Researchers at the Lawrence Berkeley National Laboratory have discovered a new mechanism by which a photovoltaic effect can be obtained in thin layers of a semiconductor
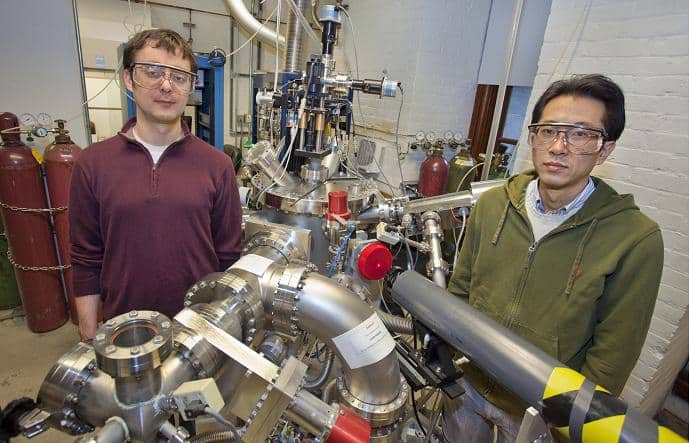
An innovative method of converting solar energy into electricity, recently discovered, could illuminate the future of photovoltaic technology. Researchers at the Lawrence Berkeley National Laboratory have discovered a new mechanism by which a photovoltaic effect can be obtained in thin layers of a semiconductor. This innovative route to energy production overcomes the band-gap limitation in electrical voltage, a limitation that continues to slow the development of efficient solid-state solar cells.
During the researchers' work with bismuth ferrite, a ceramic material composed of bismuth, iron and oxygen that is multiferroic, that is, it simultaneously exhibits both ferroelectric and ferromagnetic properties - they discovered that the result of photo- Voltaic can appear independently at the nanometer level due to the particular crystalline structure of the material - distorted rhombohedral. In addition, they demonstrated that activating an electric field on the material allows the researchers to change this crystalline structure and thus control its photovoltaic properties.
"We are excited to find previously unobserved functionality at the nanometer level in a multiferroic material," said Jan Seidel, the physics researcher who conducted the study. "We are now working on utilizing this idea for devices related to high-efficiency energy research." The research findings were published in the scientific journal Nature Nanotechnology.
At the heart of solid state type solar cells is a pn junction, the interface between the semiconductor layer that is filled with positively charged "holes" (niches from which electrons were removed), and a layer that is filled with negatively charged electrons. When photons are absorbed from the sun's radiation, the energy contained in them creates electron-hole pairs that can separate in a region known as the "depletion zone", a microscopic complex in a pn junction that is only a few micrometers in size, and then be collected as electricity. However, in order for this process to occur, the photons They must penetrate the material and reach the deficiency region and their energy must match exactly the energy of the band gap of the semiconductor - the energy gap between the conduction band and the valence band.
"The maximum voltage that conventional solid-state photovoltaic devices are capable of producing is equal to the energy of their electronic band gap," explains the researcher. "Even for so-called serial cells, where several semiconductor pn junctions are connected together, the voltage is still limited due to the finite penetration depth of light into the material."
The researchers discovered that by projecting white light onto bismuth ferrite, a material that is both proelectric and antiferromagnetic, they can produce photovoltaic voltage from submicroscopic complexes with a diameter between one and two nanometers. This voltage was significantly higher than the electronic band gap of the material.
"The band-gap energy of bismuth ferrite is equal to 2.7 volts. From our measurements we know that using the mechanism we discovered we are able to produce a voltage of about 16 volts over a distance of 200 microns. Moreover, this voltage is mainly directly proportional to the distance, that is - greater distances could lead to receiving higher voltages."
Behind this state-of-the-art mechanism for producing photovoltaic voltage are boundary walls - two-dimensional layers that cross the material and serve as transition zones that separate different complexes with ferromagnetic and ferroelectric properties. In their research, the scientists found that these boundary walls can be used for exactly the same purpose as the depletion regions for the hole-electron separation and have unique advantages.
"The very tiny dimensions of these demarcation walls make it possible to stack a large number of them in a layered array, side by side, so that the sun's radiation still reaches them," explains the researcher. "As a result, it is possible to increase the photovoltaic values far above the electronic band gap of the material."
The photovoltaic result is obtained because in the boundary walls the polarization direction of bismuth ferrite changes, a fact that affects the electrostatic potential. Through annealing treatments of the substrate on which the bismuth ferrite "grew", its rhombohedral crystals can be influenced to produce boundary walls that change the direction of the electric field polarization by: 71, 109 or 180 degrees. The researchers measured the voltage obtained by changing at 71 and 109 degrees.
The researchers were also able to use an electrical pulse at a voltage of 200 volts to reverse the polarity of the photovoltaic output or even completely cancel it. Such controllability of the photovoltaic result has never been reported for conventional photovoltaic systems, and this capability paves the way for the development of new applications in the fields of nano-optics and nano-electronics.
"Although we have not yet demonstrated these possible new applications and devices, we believe that our research will advance ideas and thoughts based on this new direction of photovoltaic effect," adds the lead researcher.