When wandering in a square almost empty of people, it takes a very short period of time to reach the person standing on the other side of the square. This is also the case in the journey of proteins inside the cell
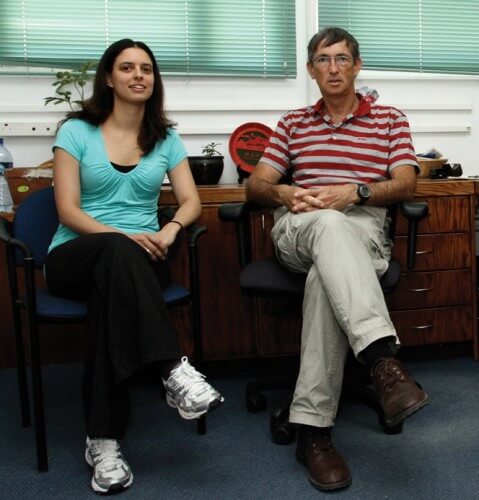
When wandering in a square almost empty of people, it takes a very short period of time to reach the person standing on the other side of the square. Conversely, when the square is crowded with people, how much more time will it take to reach a certain person?
A similar question is posed by biochemists who study proteins. They are able to easily observe two proteins in vitro, and measure how long it takes for them to meet and bond in solution. This case is similar to the empty square, but in practice, the inner space of the cell is very crowded: in order for the proteins to meet and interact, the proteins must find their way in a dense mass of different molecules in the cytoplasm of the cell. To try to bridge the gap between the test tube and the living cell, scientists usually add different proteins, or protein-like substances, to the test tubes in which the experiment is carried out.
To what extent does the dense space of the cell affect the rate at which interactions between proteins take place? To answer this question, Prof. Gideon Schreiber, research student Yael Philip, and Dr. Vladimir Kish, from the Department of Biological Chemistry, developed a method to directly observe proteins found in living cells. Using the method, they were able for the first time to measure the rate of interaction between proteins inside a living cell. Their findings were recently published in the journal of the US National Academy of Sciences (PNAS).
To measure the rate of interaction, the scientists activated individual cells, causing them to produce a certain protein. They gently inserted the second protein into the cell using a microscopic needle, and immediately began measuring. The two proteins were marked with a special fluorescent substance, which glows during the transition of energy - thus signaling an interaction between the proteins.
To their surprise, the researchers discovered that the rate of interaction between the proteins in the study was only slightly slower than that of proteins found in solution, in a test tube. Even when the scientists created mutations in the proteins - which made them act faster or slower - no significant differences were still seen.
Prof. Schreiber and the members of his group hypothesize that the "crowded crowd" scenario, in a seemingly paradoxical way, may explain why the interactions between proteins in the compressed cytoplasm occur faster than expected. "In the beginning, the density does indeed delay the progress of the proteins," says Prof. Schreiber. "But when they get close to each other, the busy crowd actually pushes them to meet. The proteins do not automatically 'know' each other - they may collide many times before any interaction takes place. The jumps and pushes between different molecules in this dynamic crowd increase the number of random meetings between the two proteins, which increases the chance of interaction between them."
The new findings imply that, despite the difference between the test tube and the living cell, the results of experiments carried out in test tubes roughly correspond to what is done in cells. However, the new method developed in Prof. Schreiber's laboratory may advance the study of the molecular interactions between proteins in their natural environment: living cells.
Prof. Schreiber: "For the first time, we were able to measure the rate of interaction between molecules within a single living cell. Thanks to fluorescence imaging technology, we are able to see the actions in real time, and follow not only their rate, but also the changes in the concentrations of molecules over time. We discovered that it is even possible to focus on specific areas within the cell body. In the future, we plan to apply this method in various biomolecular studies, while collaborating with other scientists - who have already expressed interest in it."
Interrelationships between proteins in the cell. Bottom left: Light microscope photograph of the injection of the protein into the cell. Top left: the energy releasing protein (in green). Top right: When there is an interaction between the proteins, energy is transferred to the "recipient protein", which causes it to glow red. Top right: combined image of the removed protein and the receiving protein