Like many artificial devices, living things also use electrical voltage changes to power their bodies. A new study sheds light on the phenomenon and offers some ideas for innovative drugs to treat pain, neurodegenerative diseases of the nervous system and muscle diseases
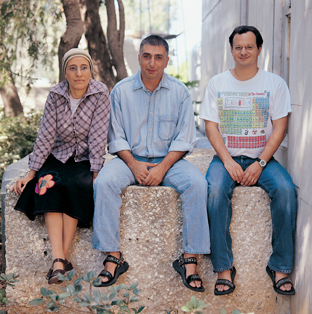
Like many artificial devices, living things also use electrical voltage changes to power their bodies. The brain, muscles, heart and senses - all make use of electrical signals. A team of scientists from the Weizmann Institute of Science, led by Prof. Eitan Reuvani from the Department of Biological Chemistry, focused on the sequence of events leading to the activation of electrical signals in the nerve cells.
One of the steps in this process is the activation of certain ion channels, which are responsible for transferring substances from outside the cell into it, and from it out. These channels are actually proteins located on the nerve cell membranes. Closing and opening these channels allows cells to generate and transmit electrical signals. Prof. Reuvani and research students Inbal Rivan and Shahar Iyonir studied the potassium channels, the opening of which causes the delay of electrical signals in nerve cells. These channels are connected to another protein located in the cell membrane, called G protein.
The channels open, the signaling stops
The activation of this protein causes the ion channel to open, which stops the electrical signaling. G protein activation is done by another protein, which is also located in the cell membrane. This protein is a receptor, which receives the instruction to act and perform its task from a nerve mediator (neurotransmitter) that binds to it. But, these neurotransmitters transmit many different types of messages, including both activation commands and commands to inhibit the electrical signals. When the G protein is activated, it affects a variety of cellular processes, but in practice, activating a certain receptor by binding the appropriate mediator results in the selective activation of a defined mechanism in the cell, for example potassium channels. How is this choice made? How does the receptor "know" that it must activate the G protein that opens the ion channel and stops the electrical signaling? How does this chain of precise, fast and selective actions work?
Prof. Reuvani and the members of his research group discovered that the proteins that make up the system are not located separately on the cell membrane, but are connected to each other and form a common structure, even before they are activated. This organization ensures that when the signal arrives to activate the canals, the entire process can be regulated and quickly controlled between the "neighbors". This enables both selectivity (the receptor knows in advance which G protein it must activate), and precise timing. The difference between the resting state of the system and the activated state is due to the three subunits that make up the G protein.
The main unit is bound to the receptor, and the other two are bound to the ion channel, thus forming a linking chain. Activation of the G protein by the receptor changes the relative position of the subunits within the co-structure, causing the channel to open. The evidence for the existence of this operational array was obtained through a technology called FRET (fluorescence resonance energy transfer), which enables the measurement of the distance between two molecules. When two molecules are close to each other, one of them, the "donor molecule", transfers energy to the "recipient molecule", thus causing the recipient molecule to emit light. The efficiency of energy transfer depends on the distance between the two molecules.
The researchers noticed that a lot of energy is transferred between the G protein and the ion channel even when the system is at rest, and concluded that they are very close to each other, in a manner consistent with the concept of a common structure. Mutations in ion channels are involved in the development of diseases and unwanted effects such as epilepsy, chronic pain, neurodegenerative diseases of the nervous system and muscle diseases, therefore these channels are a good target for drug therapy.
For example, providing an artificial replacement for a neurotransmitter that increases the activity of the channels may help treat diseases such as epilepsy, pain, hypertension and stroke. The researchers hope that these findings will lead in the future to the development of advanced ways to treat various nerve diseases.