Understanding the mechanism - which is based on a single amino acid far from the active site of the enzyme - solves a forty-year-old mystery regarding the precise activity of this enzyme
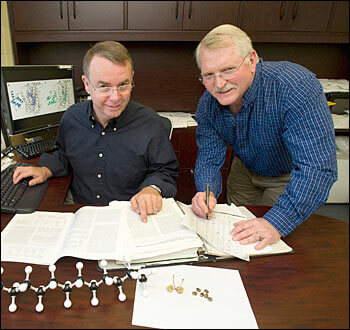
Scientists from the US Department of Energy and their partners from a Swedish research institute have deciphered how an enzyme "knows" where to insert a double bond in the case of desaturation from plant fatty acids. Understanding the mechanism - which is based on a single amino acid far from the active site of the enzyme - solves a forty-year-old mystery regarding the precise activity of this enzyme.
The research, which was recently published in the scientific journal Proceedings of the National Academy of Sciences, may lead to finding new ways to engineer vegetable oils as renewable alternatives to the oil industry.
"Plant fatty acids are an estimated $XNUMX billion market annually," said biochemist John Shanklin, lead author of the paper describing the study. "Their properties, and consequently their possible uses and value, are determined according to the position of the double bonds in the hydrocarbon chains that make up their skeleton. Therefore, the ability to control the positions of the double bonds will allow us to produce pre-adapted fatty acids that will be useful as industrial raw materials."
The enzymes responsible for the creation of a double bond, called desaturases, remove hydrogen atoms and thus produce double bonds between adjacent carbon atoms in defined positions on the hydrocarbon chains. However, the mechanism by which a certain enzyme knows how to produce a double bond in a defined position while a different enzyme close to it in its function, produces a double bond in a different position, remains unknown. "Most enzymes recognize specific characteristics that exist in the molecules with which they react, and these characteristics are very close to the site where the enzyme's activity occurs. However, all the carbon-hydrogen groups that make up the skeletons of the various fatty acids are very similar to each other and lack distinguishing characteristics," explains the lead researcher. In describing his research group's long journey to solve this mystery, the lead researcher quotes Nobel Laureate Konrad Bloch, who announced more than forty years ago that this precise removal of hydrogen "seems to push the limits of the amazing abilities of enzymes."
The researchers approached the solution of the problem by studying two genetically similar enzymes from the group of desaturases that operate in different positions: a desaturase enzyme from the castor plant that creates a double bond between carbon at position 9 and carbon at position 10 in the chain ('delta-9' desaturase); and a desaturase enzyme from the ivy plant that creates a double bond between carbon at position 4 and carbon at position 5 in the chain (delta-4); The researchers assumed that it would be possible to easily track any changes caused in these very different examples.
However, early attempts to find a plausible explanation - which included atomic-level analyzes of the crystal structures of the two enzymes - provided only a few clues. "The crystal structures are almost identical," notes the lead researcher.
The researchers' next step was to observe the binding phase of the two enzymes to their substrate - chains of fatty acids bound to a small carrier protein. First, the scientists analyzed the crystal structure of the desaturase enzyme from the castor plant bound to its substrate. In the next step, they used computer models to further investigate the "docking" of the carrier protein to its enzyme. "The results of the computerized docking model exactly matched the obtained crystal structure, which allowed the carbon atoms in positions 9 and 10 to be located exactly in the active site of the enzyme," explained the researcher.
In the next step, the scientists prepared a model for docking the carrier protein to the desaturase enzyme from the ivy plant. For this model, a different orientation was obtained that placed the carbon atoms in positions 4 and 5 in the active site of the enzyme. "So the docking model predicted a different directionality that accurately explained the specificity of the two enzymes," explains the researcher.
In order to accurately identify what was the factor responsible for the change in binding, the scientists examined the amino acid sequence of the two enzymes which included 360 building blocks. They discovered amino acid positions that distinguished the two closely related enzymes, delta-9 desaturase and delta-4 desaturase, and focused on those positions likely to react with the substrate, based on their position in the structural models.
The scientists located one such location, away from the active site, that the computer model indicated was responsible for a single amino acid change causing a change in the orientation of the bound fatty acid relative to the active site. Does this remote position of the amino acid control the position of the formation of the double bond?
In order to test this explanation, the scientists engineered a new desaturase enzyme, in which the amino acid aspartic acid normally present at this position in delta-9 desaturase was replaced by the amino acid lysine present in delta-4 desaturase. The result: an enzyme that was exactly like delta-9 desaturase except for the fact that it is capable of producing a double bond precisely at the delta-4 position. "It is quite exciting to see that changing only a single amino acid can cause such a far-reaching effect," the researcher notes.
The computer modeling helped explain why: it showed that the negatively charged aspartic acid in delta-9 desaturase repels a negatively charged region on the carrier protein, leading to a binding directionality that favors the delta-9 position; Conversion of this acid to the positively charged amino acid lysine leads to an attraction between the enzyme and its carrier protein, which gives rise to a binding directionality that favors the delta-4 position.
Deciphering this mechanism led one of the researchers to add a second positive charge to the delta-9 desaturase enzyme with the intention of further strengthening the degree of attraction. The result was an almost complete switch from a castor plant-derived enzyme with a double bond of the delta-9 type to the other delta-4 type, a result that adds compelling support to the hypothesis of remote control.
"Thanks to our new understanding of this mechanism, I am optimistic that we will be able to produce new enzymes that will have the desired specificity in order to produce innovative fatty acids in plants. These can be used as raw materials for the preparation of renewable fuels," adds the chief researcher.
The news about the study