We expect that in the future all medicine will operate according to these principles (similar to measuring sugar), but already in cancer research you can find examples of using miniaturized technology to generate the information necessary to observe the disease from a comprehensive systemic perspective.
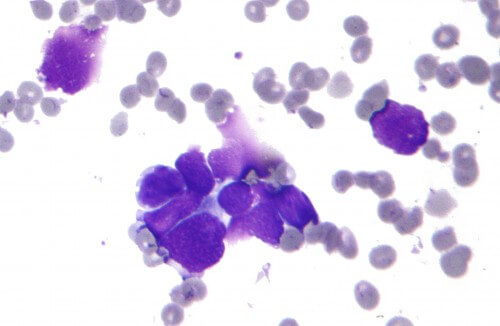
A diabetic who devoured cakes at an office party, or who is going to work out at the gym, can use a portable measuring device to perform a quick blood sugar test, then adjust the amount of insulin or food he consumes to avoid a spike or drop in his blood sugar level. And anyone who believes that the small and inexpensive test device, which allows diabetics to check their glucose level during the day, is a matter of convenience and nothing else - surely would not have been a diabetic ten years ago or more, when life alongside this disease was accompanied by many more fears and guesswork and much less control.
This cheap and convenient technology for extracting information from the body, and the enormous improvement in the quality of life it gives to patients, is an example of the future nature of medicine as a whole: it foresees the future from happening and prevents it, is more adapted to the personal needs of the patient and provides tools that allow him to take part in maintaining his health. In fact, we believe that medicine is already moving in this direction, mainly thanks to new technologies that make it possible to measure and analyze biological information quickly and cheaply.
One of the keys to this revolution in medicine is the extreme miniaturization of technologies for the purpose of extracting diagnostic information from tiny amounts of blood, or even single cells taken from diseased tissues. The tools being developed today, whose dimensions are measured in microns and nanometers (millionths and billionths of a meter), are able to measure a large number of biological molecules quickly and with great accuracy, and all this at a future cost of a few cents per measurement. The combination between low cost and high capabilities opens new paths for research and treatment of diseases by observing the human body as a dynamic system of molecular reactions. These systemic measurements are fed into computational models, and these can reveal early signs of a problem. Combining these insights with new nanotechnological methods for healing allows the treatment to be focused on the problem, and the problem only, thus preventing serious side effects.
We expect that in the future medicine as a whole will operate according to these principles, but already in cancer research you can find examples of using miniaturized technology to generate the information necessary to observe the disease from a comprehensive systemic perspective.
systemic medicine
To develop a computer model of a system huge amounts of data are needed. Living beings contain a great deal of "digital" information that can be measured, quantified and programmed into the model. This biological information begins in the creature's genetic code. Each and every cell in the human body carries a complete copy of the human genome, which consists of three billion pairs of DNA bases, which are the letters of the genetic alphabet. These "letters" are encoded by approximately 25,000 genes, which represent instructions for activating cells and tissues. Inside each cell, the genes are transcribed into more mobile forms, into discrete segments of messenger RNA that carry the instructions to cellular equipment that reads the RNA and produces chains of amino acids according to the coded instructions. The amino acid chains fold into proteins, which are actually three-dimensional molecular machines that carry out most of life's functions.
Within a biological system, such as a human body, all this "data" is transmitted, processed, assimilated and finally translated into activity by networks of proteins that interact with each other and with other molecules within the cell that have biological importance. Looking at the entire system as a network of these related events, disease can be seen as the result of some disruption in the network's normal programmed information patterns. The initial cause may be a defect within the system, such as a random change in the DNA causing a change in a coded instruction, or even some environmental influence penetrating the system from the outside, such as ultraviolet light from the sun that may cause damage to the DNA that over time will lead to skin cancer. As the effect of the initial disorder expands, the patterns of information continue to change, and these dynamic changes in patterns mechanistically explain the nature of the disease [see figure on next page].
Building an accurate computer model for this kind of biological network is a huge effort. The task requires computational integration of millions and even more measurements of messenger RNA and protein levels to fully describe the dynamics of the system's transition from health to disease. However, an accurate model that can correctly predict the results of disruptions could lay the groundwork for a revolutionary change in the perception of health and illness and in the way we approach them medically.
In the last decades, for example, cancer is the most studied disease, but the traditional characterization of tumors is done quite roughly, according to the size, the location in a certain organ or tissue, and whether the malignant cells have spread from the primary tumor. The more advanced the cancer is according to these diagnostic "stages", the bleaker the patient's prognosis. However, even this accepted procedure suffers from many contradictions. Patients who were diagnosed with the same cancer, and received similar radiation and chemotherapy treatments, sometimes react very differently - some recover completely and some deteriorate rapidly.
Measurements of messenger RNA and protein concentrations in cancerous tissues revealed the deficiencies in these traditional approaches, and showed that two apparently identical cancerous tumors are, in fact, the result of network disruptions that differ from each other in the purpose of the change. Based on such molecular analysis, many types of cancer that were previously considered to be one disease are now recognized as different diseases.
Let's take prostate tumors as an example. About 80% of them grow so slowly that they will never cause any damage to the body that houses them. The remaining 20% will grow faster, invade the tissues around them, and even send metastases to distant organs and eventually lead to the death of the patient. Our research group is now trying to identify the disrupted biochemical networks in prostate cells that characterize each of these two types of cancer so that it is possible to know in the first place which type the patient has. This information could save 80% of the patients from unnecessary surgery, radiation or chemotherapy, and needless to say pain, urinary leakage and impotence accompanying these treatments.
We also analyze the molecular networks within the prostate gland that allow us to distinguish between subtypes among those 20% of violent cases where treatment is necessary. For example, by analyzing the networks characteristic of early and metastatic prostate tumors, we identified a protein secreted into the blood that appears to be an excellent marker for metastatic cancer. Tools such as this can segment a given disease such as prostate cancer into precise subtypes and allow the doctor to intelligently choose the appropriate treatment for each and every person.
Disease detection
These analyzes of messenger RNA and proteins from cancer tissues provide information about the nature of a known cancer, but the power of the systemic approach is also great to help distinguish between healthy and diseased. The blood passes through all the organs of the body and carries proteins and other molecules from them, so it serves as an excellent window into the entire body system. The ability to discover an imbalance in proteins or in certain types of messenger RNA could therefore be used to warn of the presence of a disease and locate its place in the body and its nature.
Our research group approached the challenge of assessing the condition of the entire body system according to the blood picture by comparing populations of messenger RNA from the approximately 50 different organs in the body. We found that in every organ of the human body there are 50 or more types of messenger RNA that are mainly produced in that organ. Some of those types of RNA carry the code for proteins unique to the organ that are secreted into the bloodstream, and their level will therefore reflect the activity of the networks that regulate their production within the organ. When these networks malfunction due to disease, the levels of the corresponding proteins change. These changes should allow us to identify the disease, because each disease in an organ will disrupt specific biological networks in unique ways.
If we can measure the level of about 25 proteins from each of these organ-specific fingerprints, we can detect all diseases by determining which networks have gone wrong with just a blood test. Apart from early detection, which is so important in cancer, this approach will make it possible to separate the disease into its various sub-issues, to monitor its progress and then its response to treatment. Initial proof of this principle was obtained by monitoring the development of prion disease in mice.
We injected mice with infectious prion proteins that cause a degenerative brain disease, similar to "mad cow disease", and then analyzed the entire messenger RNA population in the brains of the infected animals and a control group at 10 different time points during the development of the disease. From these data we identified changes in 300 types of messenger RNA that encode the response to prion disease. About 200 of them belonged to four biological networks that explained all known aspects of the disease, and about 100 others referred to aspects of the disease that were not known until then. During the research on the disrupted networks we also identified four blood proteins that indicated the existence of prion disease even before any symptoms were observed. These proteins can be used as markers for early diagnosis, pre-symptomatic, and the benefit of this for preventive medicine is obvious.
As part of these studies, 30 million measurements were taken, and we developed a series of software to analyze, integrate and model these huge amounts of data. Building models for molecular networks capable of predicting diseases and translating these models into useful tools for medicine will require fast, sensitive, and most importantly - cheap methods for determining the DNA sequence and measuring concentrations of messenger RNA and proteins.
Measure molecules
Many scientists have noticed that the technological progress in determining the DNA sequence is conducted according to Moore's Law known in the development of computer chips. The law says that the number of functional components that can be put on a chip per unit cost has doubled every 18 months for the past decades. In fact, next-generation DNA sequencing devices increase the speed of DNA reading at a much faster rate than Moore's Law. For example, sequencing the human genome for the first time probably took three to four years and cost about $300 million. We believe that within 5 to 10 years, sequencing a human genome will cost less than a thousand dollars, a 300,000-fold decrease, and will be done in one day. Similar progress in other relevant biomedical technologies will allow the development of preventive and personalized medicine in the next ten years.
Today, a blood test that measures the level of a single diagnostic protein, such as prostate-specific antigen, costs the hospital about $50. Since in systemic medicine it will be necessary to measure a large number of such proteins, the cost must decrease considerably. The measurement time is also included in the cost. Today, a blood test takes from a few hours to a few days, partly because of the many steps required to separate the blood into its components - cells, plasma, proteins and other molecules - before each of them can be measured with tests of varying accuracy.
Extreme miniaturization may greatly increase the accuracy and speed of measurements compared to current technologies. Several micro and nano technologies are already proving their value as a research tool for collecting the necessary data to obtain a systemic view of biological information. However, in order for it to be possible to adopt the systemic approach to routine treatment of patients, the cost of each protein test needs to drop to a few cents - a goal that is unlikely to be achieved by many of the developing nanotechnologies.
Two of us (Heath and Hood) developed a chip, four centimeters wide, that tests protein levels in a drop of blood using a very miniaturized version of the conventional detection methods. The chip is made of glass, plastic and chemicals only, so its production cost is very low. Our device takes 2 microliters of blood, separates the cells from the plasma and measures the level of a dozen plasma proteins within a few minutes. Right now, the estimated cost of using the initial version is maybe 5 to 10 cents per protein, but once development is complete, this technology should meet the cost requirements of systemic medicine.
Expanding the measuring capacity of the chip to hundreds of thousands of proteins will take time, but the progress in the design of microscopic flow systems, in the chemistry of surfaces and in the science of measurement is quickly bridging the gap between what can be done today and what will be required to fully realize a new and personalized medicine that advances medicine to Mecca. Our colleagues at the California Institute of Technology, Steven R. Quick and Axel Scherer, for example, have developed a microscopic flow system that combines valves and pumps on a chip. Their miniaturized piping allows chemicals, molecules and biological samples to be very precisely directed to each of the many cells on the chip, with a separate and independent measurement being performed in each cell. Thus they turn a lab-on-a-chip into many labs-on-a-chip, and offer a way to further reduce the costs of biological measurements.
The implications of miniaturized technology for preventive care are equally important. From new insights into the diseased networks may grow new targets for new medical treatments that will restore the dynamics of the networks to repair. In the short term, the systemic view may help more effectively direct existing drugs to their target by adjusting the optimal combination of drugs for each patient. Nanotechnology can also significantly reduce the dose of drugs needed to treat cancer.
Small and purposeful
Nanoscale healing agents are relatively small compared to most things but large relative to a molecule, and their size range provides an unprecedented level of control over the behavior of drug particles within the body. The size of nanometer particles can be between 1 and 100 nanometers, and they can be produced from a variety of drugs on the market, such as chemotherapy agents or gene silencing RNA strands (siRNA).
These charges can be trapped inside capsules of synthetic materials such as polymers or fat-like molecules, and to the surface of the particle can be added causative factors such as antibodies and other molecules designed to bind to specific cell proteins. The modularity gives the nanometer drugs versatility and the ability to perform complex activities in the appropriate place and at the appropriate time within the patient's body.
One of the biggest challenges in developing and using cancer drugs is introducing them to the diseased tissues without poisoning the entire body. The size alone gives treatments with nanometer particles, even the simplest ones, special properties that determine the movement of the particles to and within the tumors. Nanometric particles smaller than 10 nanometers are cleared from the body quickly through the kidneys, while particles larger than 100 nanometers have difficulty moving within the tumor. Particles in the range between 10 and 100 nanometers wander through the body with the bloodstream. They cannot, normally, penetrate into the healthy tissues through the walls of the blood vessels, but the blood vessels of cancerous tumors are abnormal and their walls are strewn with large pores, through which the nanometer particles can penetrate into the tumor tissue. Due to this feature, nanometer particles tend to accumulate in tumors and do not harm other body parts, thus avoiding the severe side effects of conventional cancer drugs.
Even if a normal drug manages to reach the tumor cells, pump proteins in the cell may remove it from the cell even before it has had time to take effect - this is a common mechanism of drug resistance. Nanosized particles enter the cell in a process called endocytosis. This is a natural process that surrounds foreign objects in a membrane to bring them into the cell, and the pocket protects the particle's charge from the cellular pumps.
Certain cancer drugs, now reclassified as nanosized particles, have been in use for some time and demonstrate some of the fundamental advantages of nanosized particles in entering cancer cells and minimizing damage to healthy tissues. Liposomal doxorubicin, for example, is a common chemotherapy drug that has been wrapped in a lipid membrane and used in this way to treat ovarian cancer and multiple myeloma. Doxorubicin damages the heart, and its lipid-wrapped version damages the heart much less. However, a new side effect was observed - skin toxicity.
New nanometer particles, for example, the one called IT-101, which has already passed the human safety tests in the first phase of clinical trials, have a more complex structure that gives them multiple functions. IT-101 is a 30 nanometer diameter particle composed of polymers attached to the drug camptothecin, a small molecule drug very similar to two chemotherapy drugs approved by the US Food and Drug Administration: irinotecan and topotecan. While camptothecin alone does not stay in the blood for more than a few minutes, the IT-101 particles are designed to travel in the bloodstream and stay there for more than 40 hours. The prolonged stay in the blood gives IT-101 time to penetrate and accumulate in tumors. The particles enter the tumor cells and release the camptothecin slowly, so that its effect is intensified. While the drug is released, the rest of the particle, made of polymer, breaks down into small units that are excreted through the kidneys without leaving a trace.
In the clinical tests, the researchers were able to reach drug doses that preserved the patients' quality of life without causing the side effects, such as vomiting, diarrhea and hair loss, typical of chemotherapy, and without new side effects. The high quality of life during the treatment is very exciting, and although the first phase of the tests focuses on safety, evidence was also found that the drug worked well against the disease. This is encouraging, because the patients participating in the first phase trials are those who have previously undergone many conventional treatments that failed. At the end of the six months of the experiment, some of the participants continued to take the drug with special approval (given before FDA approval to patients in a serious condition for which there is no other way to treat them), and among the survivors after a year are patients in advanced stages of lung, kidney and heart cancer.
Because the side effect profile of this drug is so low, it will now be tested in phase II clinical trials (testing effectiveness) in women after ovarian cancer chemotherapy. Instead of "waiting and seeing" if the cancer spreads, IT-101 will be given as a maintenance treatment in hopes of preventing the disease from progressing. The results of the IT-101 trials and similar encouraging news from trials of other nanoparticle-based therapies are beginning to paint a picture of what well-designed nanomedicine might offer. Indeed, the next generation of synthetic nanometer particles, which are much more sophisticated, reveals to us a touch of the true potential of this technology and teaches us about the future contribution of these drugs to a systemic view of the disease and its treatment.
The pharmaceutical company Clando, from Pasadena, California, began clinical trials in 2008 with a messenger RNA transport system invented by one of us (Davis). This well demonstrates the new approach: proteins attached to the surface of the particles target certain receptors found in high concentrations on cancer cells. The particles penetrate the cells and release messenger RNA molecules there, which have been prepared to match a certain desired gene, and these inhibit the production of the proteins encoded in the gene.
However, this multifunctional nanometer medicine is only the beginning of the story. Once we understand the principles of action of nanoscale particles in humans, we will be able to apply the idea to healing systems that will carry combinations of drugs that will be released, each one, at a personalized rate. For example, if we want to inhibit a protein that disrupts the activity of a certain drug, we can create a particle that will first release a messenger RNA that inhibits the gene for this protein, and only then will it release the drug molecules. As our understanding of the molecular changes in the transitions between health and disease deepens, a part of the nanometer particle approach in the treatment of diseases at the molecular level will certainly increase.
The big picture
The systemic approach to the disease is based on the idea that the analysis of the dynamic networks that the disease disrupts and the detailed mechanistic understanding of the disease state that emerges from this can change from end to end all aspects of medicine: more accurate diagnoses, new and more effective approaches to treatment, and even prevention. The systemic biology approach to diseases promotes the development of many new technologies, including microscopic flow, nanotechnologies, new measurement and imaging devices and computational innovations capable of analyzing and merging large amounts of biological information and building a model based on them.
In the next ten to twenty years, at least two new approaches will revolutionize medicine's ability to personalize and predict. Determining a personal genome sequence will make it possible to determine the future state of a person's health with increasing precision. Inexpensive measurements of proteins in the blood will make it possible to follow, in an ongoing and comprehensive manner, the changes in a person's health.
Preventive medicine begins with the identification of proteins within a diseased network, with the help of which it is possible to correct the behavior of the network and which will lead, over time, to the development of preventive drugs. For example, a woman at increased risk of ovarian cancer, who at age 30 will start taking a nanometer drug specially designed to neutralize the molecular source of the risk, may lower her chances of developing ovarian cancer during her lifetime from 40% to 2%.
Such knowledge about the source of health and disease will also make it easier for people to take part in decisions concerning their health, just as today diabetics have tools and information that help them control the quality of their daily lives.
Preventive medicine with predictive ability, which is personally adapted to allow a person to take an active part in maintaining his health, will bring about far-reaching changes in our society. The health industry will have to fundamentally change its business plans, which currently do not produce drugs that are highly effective and low cost. Emerging technologies will also lead to the digitization of medicine, which means the ability to extract disease-relevant information from a single molecule, individual cells, or individual human beings, just as information and communication technologies were digitized 15 years ago. Thanks to the new technologies, which are high-yield and low-cost, the cost of medical treatment should drop sharply, so that it will be accessible to everyone, even in developing countries.
In the next ten years we expect the realization of some exciting promises in the field of cancer. One is blood tests for pre-symptomatic diagnosis, which will catch the tumors at an early enough stage for conventional treatment. Another promise is the classification of malignant tumors according to the type of tumor, and not necessarily according to the tissue in which it is found, which will make it possible to treat it with the most appropriate drugs. Also, identification of disrupted networks will enable the accelerated development of drugs that will be cheaper and much more effective. This new approach to medicine therefore has the potential to revolutionize healthcare that will affect us all.
About the authors
James R. Heath (Heath) is the director of the Systems Nanobiology Center for Cancer Research and a professor of chemistry at the California Institute of Technology (Caltech), where he works on nanoscale materials and nanoelectronic circuits, as well as technologies for cancer diagnosis and treatment. Mark A. Davis (Davis), a professor of chemical engineering at Caltech, develops unique materials for experimental treatments and founded two companies, Insert Therapeutics and Calando Pharmaceuticals, which develop nanometer drugs. Leroy Hood is the president of the Institute for Systems Biology in Seattle, which he founded after developing pioneering technologies for sequencing and synthesizing DNA and proteins and founded several companies, including Amgen, Applied Biosystems, Systemix, Darwin and Rosetta. Hood and Heath also founded Integrated Diagnostics, a systems medicine company that searches for biomarkers of disease and develops microscopic flow devices and nanotechnologies to turn these markers into diagnostic tools.
And more on the subject
NanoSystems Biology. James R. Heath et al. in Molecular Imaging and Biology, Vol. 5, no. 5, pages 312-325; September/October 2003.
Nanotechnology and Cancer. James R. Heath and Mark E. Davis in Annual Review of Medicine, Vol. 59, pages 251-265; February 2008. (First published online: October 15, 2007.)
Nanoparticles Ttherapeutics: An Emerging Ttreatment Modality for Cancer. Mark E. Davis et al. in Nature Reviews Drug Discovery, Vol. 7, no. 9, pages 771-782; September 2008.
Integrated Barcode Chips for Rapid, Multiplexed Aanalysis of Proteins
in Microliter Quantities of Blood. Rong Fan et al. in Nature Biotechnology.
Advance online publication: November 16, 2008.
One response
I haven't read all the articles on nanoscale cancer treatment and I'm not a chemist or a doctor, but I've been wondering for some time why cancerous tumors haven't been treated by injecting relatively small amounts into the tumor's immediate surroundings using imaging techniques such as ultrasound or even old x-rays from before surgery.