Continued from Part A - The 2016 Nobel Prize in Chemistry is awarded to Jean-Pierre Sauvage, James Fraser and Bernard Feringa for their development of molecular machines thousands of times the size of a human hair. This is the story about their success in connecting molecules together to create molecular machines such as a tiny elevator, motors and even artificial muscles.
An elevator, a muscle and a tiny computer chip
Since 1994, Stoddart's research group has used a number of rotaxanes to construct a variety of molecular machines, including an elevator (2004, Figure 4), capable of lifting itself 0.7 nm above its base level, and even an artificial muscle (2005).
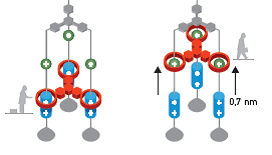
As part of collaborations with other researchers, Fraser Stoddart also developed a computer chip based on a rotaxane structure with a memory capacity of 20 KB. The transistors used today inside computer chips are admittedly tiny
But they are huge compared to molecular transistors. Researchers believe that molecular computer chips may bring about a revolution in computer technology similar to the revolution created by silicon-based transistors.
Jean-Pierre Sauvage also studied the potential of rotaxanes. In 2000, his research group was able to thread two ring molecules together to form a flexible structure resembling a single human muscle tendon (Figure 5). They also synthesized a motor-like structure, with the rotaxane ring rotating in different directions as required.
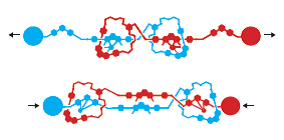
Creating motors capable of rotating continuously in the same direction is an important goal in the field of molecular engineering. Many attempts were made in the nineties, but the first to achieve this was the Dutch researcher Bernard Feringa.
Ben Feringa builds the first molecular motors
Just like Fraser Stoddart, Ben Feringa grew up on a farm and was drawn to chemistry for its endless creativity. As he expressed in one of his interviews: "It is possible that the strength of chemistry lies not only in understanding, but also in creation, in the preparation of molecules and materials that did not exist before..."
In 1999, when Ben Feringa synthesized the first ever molecular motor, he used a number of brilliant tricks designed to allow the motor to spin in one and only one direction. Normally, the motions of molecules are governed by changes; On average, an individual molecule rotates it several times left and right. However, Ben Feringa designed a molecule that was mechanically constrained to be rotatable in only one direction (Figure 6).
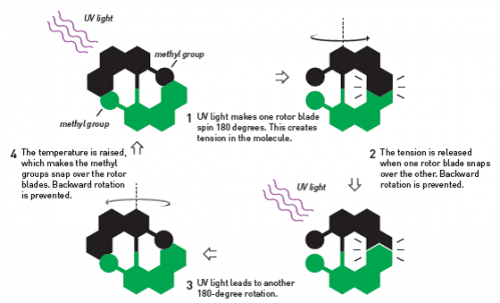
The molecule was composed of parts that resembled two small rotor blades, two flat chemical structures linked by a double bond between two carbon atoms. A methyl group was attached to each of the rotor blades; These groups, together with the rotor blades, act like a ratchet that forces the molecule to rotate in one direction only. When the molecule is exposed to a beam of ultraviolet radiation, one of the rotor blades rotates 180 degrees around the double bond. Then the ratchet moves to its fixed position. When another beam is emitted, the rotor blade rotates 180 degrees again. And so it continued, round after round, in the same direction.
The first engine was not very fast, but the Fringa research group improved its performance. In 2014 the engine was rotating at a speed of 12 million revolutions per second. In 2011, the group also synthesized a four-wheeled nanocar – a molecular chassis attached to four rotating structures that act as wheels. As the wheels turn, the car moves forward on the surface (Figure 7).
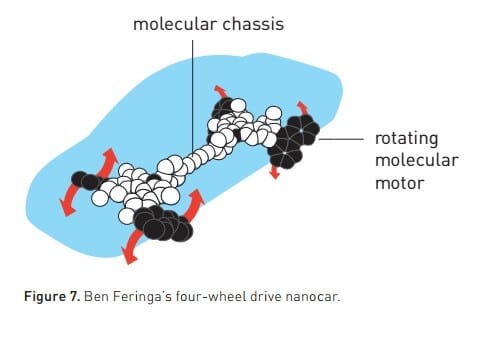
A molecular motor that rotates a small glass cylinder
In another amazing experiment, Ben Feringa's research group used molecular motors to rotate a long glass cylinder 28 micrometers in size (10,000 times larger than the molecular motors). Only one percent of the liquid crystal consists of molecular motors, but when the researchers start spinning them, the motors change the structure of the liquid crystal as they rotate. When the researchers placed the glass cylinder on top of a liquid crystal, it rotated following the movement of the motors.
Molecular Toolbox
Jean-Pierre Sauvage, Fraser Stoddart and Ben Feringa's groundbreaking steps in the development of molecular machines gave rise to tools for creating chemical structures, tools used by researchers around the world to build increasingly complex structures. One of the most amazing examples is a molecular robot that can capture and connect amino acids. Such a structure was indeed developed in 2013 and is based on the rotaxane molecule.
Other researchers have attached molecular motors to long polymers to form an intricate network. When the molecular motors are exposed to light, they shrink the polymers into a compact package. In this way, it is possible to accumulate and store energy inside the molecules, and if the researchers find a way to retrieve this energy, then it will be possible to develop a new type of battery. The material also shrinks when the motors weave the polymers, a result that could be used for the development of detectors that respond to light.
Towards a new and vibrant chemistry
An important part of the development born thanks to the winners of the Nobel Prize in Chemistry for 2016 is that researchers moved molecular systems away from a state known as equilibrium. All chemical systems strive to achieve equilibrium - an energy state as low as possible - but it can also be a dead end. You can look at life itself as an example of this. When we eat, the molecules in our body are fed by the energy stored in the food and move our molecular systems away from the equilibrium point, to higher energy levels. In the next step the biomolecules use energy to drive chemical reactions required for the body to function properly. If the body was in chemical equilibrium, we would already be dead.
Just like the molecules of life, the artificial molecular systems of Sauvage, Stoddart and Fringa perform controlled tasks. The world of chemistry, therefore, took the first step towards a new world. History has indeed shown us the revolutionary effects of the miniaturization of computers, when today we are only watching the beginning of the parallel revolution in molecular machines. In terms of stages of development, the molecular motor is in the same place as the electric motor was in 1830, when researchers proudly displayed a variety of cranks and spinning wheels in their laboratories without imagining that they were the inventors who would lead to the development of electric trains, washing machines, fans and home food processors.
And so, 32 years after Feynman's visionary lecture, today we can only guess what exciting developments are still in store for us. At the same time, we have a firm answer to his first question - how tiny would the machine be that a person could build? At least 10,000 times smaller than a human hair.