The biggest stars die in explosions that are more powerful than we ever thought. Some of them occur, among other things, following the formation of antimatter
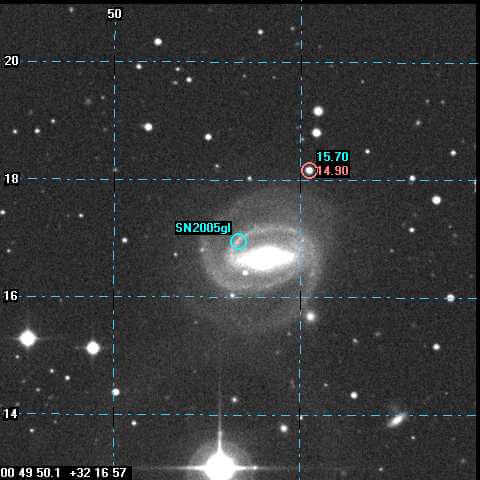
In mid-2005, the upgrade of one of the pair of giant telescopes at the V. M. Keck Observatory on Mauna Kea in Hawaii was completed. Using a system to correct the distortions caused by the passage of light rays in the atmosphere, it is now possible to take sharp pictures from the ground like those taken by the Hubble Space Telescope. Srinivas Kulkarni from the California Institute of Technology (Caltech) encouraged several young researchers from this institute - myself among them - to apply for observation time. He let us know that once the rest of the astronomical community realizes how wonderful these telescopes are, there will be a great deal of competition for every available time slot.
Following his advice, my postdoctoral fellows at the time, Derek Fox and Doug Leonard, and I decided to try to do research that had previously been done almost exclusively with the Hubble Space Telescope: the hunt for stars that had exploded as supernovae. In other words, we wanted to know what stars that are about to explode look like.
For decades astrophysicists have been able to predict which celestial bodies are about to go supernova. For example, we know that bright blue stars are expected to explode soon. But the meaning of the word "soon" among astronomers is within a million years, more or less. Therefore, although watching the entire process as it unfolds before our eyes will allow us to better understand it, we cannot afford to simply sit and patiently observe one and only star.
We thought that Mitzpe Kek could help us, and we got a single night of observation in November 2005. When I flew to Hawaii I was quite worried, and I was hoping that the weather would be nice, because it was the one and only chance to try this new approach. Fortunately, the weather cooperated. That night of observation set me on a path of research that ultimately helped overturn long-held views about the formation of large stars and how these giants die.
The accepted opinion at the time was that very large stars do not explode. Instead, they gradually lose mass as a stellar wind. In fact, most theoretical astrophysicists have argued that these strong winds do not allow stars in today's universe to reach enormous sizes in the first place, meaning that they cannot be much heavier than, say, 100 times the mass of our Sun or so.
However, as a result of our adventure in Hawaii, we slowly began to understand that stars with a mass of at least 200 solar masses do exist in the contemporary universe, and that they end their lives in the most energetic explosions in the universe. We were equally surprised to find that some of these stars explode in a very different way than anything astronomers have ever seen, a process that involves the production of antimatter at the center of the star.
Such massive stars, and possibly even larger ones, may have been the first celestial bodies formed from the primordial gas that filled the universe at the beginning of its history. The manner of their explosion therefore tells us how the elements created by those stars could spread throughout the cosmos and eventually lay the seeds of the suns, planets and humans of today.
An unlikely start
On that single night of observation, Fox, Leonard and I hoped to observe an active supernova and then find a picture of the star, before it exploded, in the Hubble image archive. We therefore had to look for a supernova that evening in one of the many galaxies photographed by the Hubble Space Telescope in the past. The difficult part of analyzing Hubble's images will be determining which star, among the billions of stars that populate each galaxy, was the star that exploded. To do this we had to measure the position of the supernova with very high precision. Before the arrival of Adaptive Optics (AO) systems like the one at the Keck Observatory, such an operation was only possible using the Hubble itself. And even then, the task was so difficult that astronomers were able to identify with certainty only three such stars.
Among the supernovae that were active at the time, we chose one named SN 2005gl. Other groups could have thought this was an unfortunate choice, and for good reason: researchers who want to study stars that exploded as supernovae usually study events within a radius of about 60 million light years from Earth, whereas this supernova was more than three times that far away: about 200 million light years from us. For us to find the source star of SN 2005gl in the Hubble images, this star had to be one of the most luminous stars ever observed. The probability of success was low, but we felt that sometimes only risky bets can yield big fruits.
And our bet paid off. After measuring the position of SN 2005gl using the Keck data, we looked at the Hubble image and saw something there that looked like a star, but we weren't sure. If it is a single star, then its brightness (about a million times greater than the brightness of the Sun) showed that it is huge: 100 times the mass of the Sun. But in view of the prevailing position, that such a heavy star would not explode at all, most astronomers would speculate that it is more plausible that the bright spot in Hubble's image comes from a cluster of smaller, less bright stars, which together create the brightness we saw. With the help of the data in our hands, we could not rule out this possibility, at the moment.
Another strange explosion
Although our results were inconclusive, I became increasingly interested in finding observational evidence suggesting the fate of the most massive stars. But science almost never walks in a straight line from asking a question to finding the answer. At that time, I switched to dealing with stellar explosions of a completely different type, known as gamma-ray bursts, for a while, when a random event in 2006 led to another surprising finding, which raised the possibility that not only could giant stars go supernova, but they might even do so in an amazing way.
This new chapter in the story began on another night at Mitzpe Kek in 2006. This time it seemed that the gods were less kind to us and the weather was terrible. I sat by the control computer and waited while the time passed hour by hour. Just when I was beginning to think that maybe the long drive here was in vain, the clouds began to clear. The sky didn't really clear, but you could see some stars. I decided to watch the brightest supernova explosion that could be seen at that time, an unusually bright event known as SN 2006gy. Robert Quimby, then a research student at the University of Texas, had discovered it eight days earlier with a telescope more than 20 times smaller than Keck's giant reflector telescopes. I was able to watch the event for 15 minutes until the clouds thickened again, and this time definitively. It seemed that all our efforts that night were for nothing.
But then, a group led by my colleague from Catac, Eran Ofek [an Israeli astrophysicist who studied at Tel Aviv University and is now a fellow at the Weizmann Institute], analyzed the data I had collected and found that SN 2006gy was the most luminous supernova explosion ever discovered (as of that time). A parallel study led by Nathan Smith, then working at the University of California at Berkeley, reached a similar conclusion. It made no sense at all. We have not known any type of supernova that could produce so much light. The supernova SN 2006gy was in a galaxy that had not been imaged by Hubble before, so we also had no way to closely study the star from which it formed. And yet, the tremendous power of the star's explosion indicated that its mass was probably at least 100 times the mass of the Sun.
We thought of several possible explanations for this level of clarity, and two of them seemed the least implausible. According to the first explanation, the great light intensity was the result of radiation emitted from a shock wave created when the remnants of the supernova explosion caught up with the slower stellar wind that the star itself emitted before it exploded and swept away that stellar wind. The second possibility we considered was radioactivity. Supernovae synthesize new elements, including radioactive isotopes, which then decay into other, more stable elements. Perhaps this huge explosion synthesized a huge amount of radioactive material, and its slow decay injected energy into the spreading cloud of stellar debris and caused the cloud to glow with fluorescent light. But what could have produced radioactive material in such a quantity that could explain such an outrageous intensity of radiation?
The last question captured our curiosity. To try to answer it, we started to review the theoretical work done in the past. We came across old and dusty theoretical articles from the late 60s, written by three young Israeli astrophysicists at the time: Gideon Rahabi [Professor of Physics at the Hebrew University of Jerusalem], Giora Shabiv [Professor of Physics at the Technion] and Zalman Barkat [Professor of Physics at the Hebrew University of Jerusalem, Nir Barkat's father, Mayor of Jerusalem]. They proposed a new way in which a star might explode.
Stars shine because their cores are dense and hot enough to allow hydrogen atoms to fuse (that is, undergo nuclear fusion) and turn into helium and heavier elements in a process that releases energy. These two variables, density and temperature, are the main ones responsible for the physical processes that take place in the core of a massive star and the process of its development. In general, over time the core becomes denser and hotter. The core then crosses threshold by threshold in fusion processes that create heavier and heavier elements. From helium to carbon at first, then from carbon to oxygen and so on. Between crossing one threshold and the next, thousands of years to billions of years may pass, depending on the speed of the effect of the nuclear combustion in the star on the temperature and pressure in its core.
Ravavi and his colleagues calculated what would happen when a very massive star, perhaps hundreds of times heavier than the Sun, reaches a stage where its core is composed mostly of oxygen. We know what happens in smaller stars: the star shrinks, and its core heats up until the conditions allow the fusion of oxygen nuclei to form silicon. But in a supergiant star, the theoretical version, the core would contract due to gravity and heat up without becoming very dense. And so instead of fusion of oxygen, something else will happen: the physicists call this the creation of pairs.
In hot enough matter, particles with high energy, such as nuclei and electrons, emit light with great intensity. The energy of the photons (light particles) is so high that the light is in the gamma ray range of the spectrum. According to Albert Einstein's famous formula linking mass and energy, E=mc2, if two highly energetic photons collide, they can spontaneously transform into pairs of other particles; In particular, they can become a pair consisting of an electron and its antiparticle, the positron. Most of the energy of the photons is converted into matter in this way. As a result, electrons and positrons exert much less pressure than the photons from which they were created: they are simply excess weight. If the core of a very massive star reaches such conditions, the pressure inside it suddenly drops, almost as if the star had a pressure relief valve. Before, it was the pressure that kept the star from collapsing under its own weight; Now the core becomes unstable and begins to shrink rapidly.
As the density skyrockets, the oxygen fusion process has finally started. Because the threshold for oxygen fusion is crossed in a collapsing core and not in a stable core, the ignition becomes an explosion: the fusion releases nuclear energy that heats the material even more, and then the fusion process is accelerated, in an unbridled chain reaction. The star can "burn" so much oxygen in such a short period of time - a few minutes - that the energy the star releases is greater than all its gravitational binding energy. Thus, while normal supernovae leave behind charred remains like a neutron star or a black hole, in this type of explosion the body completely disintegrates and scatters everywhere. All that remains is a rapidly spreading cloud, made mostly of elements synthesized in the flare storm.
Such an event is called a pair instability supernova because the stability of the star is destabilized due to the creation of electron-positron pairs. Theorists predicted that such a supernova would produce a huge amount of nickel-56 and other relatively heavy elements. Although the bonds in the nucleus of nickel-56 are strong, it is a radioactive isotope that decays in a process that eventually produces non-radioactive iron-56. We therefore reasoned that if this scenario happened in the source star of SN 2006gy, the decay of Ni-56 might explain the intense brightness of the supernova.
Although the theory of the three astrophysicists was correct, for decades the conventional wisdom was that their hypothesized process would not actually occur in nature. Theorists who study the formation and evolution of celestial bodies thought that such massive stars could not have formed in the first place, at least not in the present-day universe. And even if they did form, they would emit such strong stellar winds that they would very quickly lose most of their mass and be left unable to form cores massive enough to reach pair instability. The situation was different a little less than a billion years after the Big Bang. So the first stars may have been massive enough to explode in pairwise instability supernovae. Maybe.
Meanwhile, the record-breaking supernova, SN 2006gy, became a hit among astronomers, spurring researchers to conduct more theoretical studies and observations. Ironically, although SN 2006gy prompted us and others in the supernova community to reconsider the pair instability model, this particular event did not appear to ultimately carry the correct radionickel signature, meaning that the light did not dim over time as predicted . In a pair instability explosion, most of the light will not come from the explosion itself but from nickel 56 and other radioactive isotopes that it anneals. Radioactivity is a process that has been studied before and after, and the decay in such a process proceeds at a gradual and predictable rate. But after SN 2006gy shone for many months it disappeared almost at once, too quickly for us to assume it was driven by radioactive force. It is therefore reasonable to assume that after all it was not a supernova of the pair instability type. The second possibility we considered, that the unusual brightness of the event was due to a shock wave, became the accepted explanation. And yet, since it was so close, I started keeping an eye out for instances of couple instability.
the real thing?
A few months after the lucky break through the clouds over Hawaii, I went on vacation in Colorado. But she was soon interrupted by an email from Peter Nugent of the American Lawrence Berkeley National Laboratory. Nugent and I have just started a "trial run" of a large project we planned to search for supernovae. In his message he sent me details of a supernova with a strange spectrum, the likes of which I have never seen.
Since the atoms of each element in nature absorb and emit light at wavelengths unique to them, the spectrum of an astronomical source provides information about the chemical composition of the material emitting the light. The spectrum emitted by Nugent's object, SN 2007bi, showed that the ratios of the quantities between the elements that made it up were unusual and that it was incredibly hot.
After returning to Caltech, I continued to follow the development of this event. It emitted about 10 times more light than a typical supernova. And the amount of light faded very slowly: the source simply refused to fade, even as the days turned into weeks and the weeks into months. I became more and more convinced that this was finally an example of a pair instability supernova. But I needed more data to be really confident in my interpretation.
Throughout 2007 and 2008, I and several other colleagues continued to observe SN 2007bi with telescopes at the Palomar Observatory in California. When the light from this explosion finally began to fade, about a year after we discovered it, I asked my colleagues at Caltech, Richard Ellis and Kulkarni to observe it with the large Keck telescopes and promised in the emails I sent them that this was "the real thing."
In the meantime, I moved to Israel with my family after I got my current job at the Weizmann Institute of Science in Rehovot. In August 2008, Kulkarni and his research student Mansi Kasliwal sent me the latest spectrum of SN 2007bi. When I did an initial and rough surgery, I couldn't believe my eyes. I analyzed the spectrum again and again, but the answer was always the same: this explosion synthesized an insane amount of nickel-56, five to seven times the entire mass of our Sun. It was ten times more than we or anyone else had ever seen and exactly what you would expect from a paired instability supernova explosion. That night I paced back and forth in my apartment thinking about this find and its implications. When my wife gave me a strange look and asked what was going on, I said "I think we made a great discovery."
Credit: Ron Miller
At the end of 2008 I went to Gerching in Germany to work with Paolo Mazzelli at the Max Planck Institute for Astrophysics. Metzli is a world expert in the quantitative analysis of spectral data from supernovae, so he could examine the results of my rough analysis. He also had additional useful data obtained from another large instrument, the Very Large Telescope (VLT) at the European Southern Observatory in Chile. We sat together in Metzli's office as he ran his software. there is! The results were consistent with my previous analyses: many solar masses of nickel-56, and the presence of elements in relative proportions consistent with the predictions of the pair instability models.
Double-Take
Although I was pretty sure we had detected a pair instability supernova, when I returned to Israel I put the data aside for a few months because I was busy with another project related to the supernova that got me on this journey in the first place: SN 2005gl. When Fox, Leonard and I found its putative source star in 2005, we couldn't say for sure that it was indeed a single entity and not a cluster of stars. Now, three years later, the supernova was gone and it dawned on me that we could conduct a simple test: if our candidate wasn't the star that exploded, it should still be there. Leonard and I went back to Hubble and took another picture to check.
At the end of 2008 we were finally sure: the star had disappeared. The source of SN 2005gl was indeed very luminous and apparently also very massive, an identical twin of Eta Carinae, one of the largest blue giants in our galaxy.
The prevailing theory about supergiant stars, which held that they lose most of their mass before exploding, was therefore wrong, at least in this case. There are very luminous and massive stars that explode before losing all their mass. And if the mass-loss theory is wrong, there may also be supergiant stars that may eventually explode in a pair instability supernova.
I was now ready to re-examine SN 2007bi and look for unequivocal evidence of pair instability explosions. Together with a team of colleagues from around the world we examined the data in every way we could think of. We analyzed its spectrum in detail and examined how the amount of light emitted evolved over time. We compared the observations to old and new models of stellar explosions. Towards the end of 2009, all the evidence accumulated to a single conclusion: the most logical, almost inevitable way to explain SN 2007bi is that it is a pair instability supernova. After more than two years of research, the time has finally come to start publishing our results.
Currently we have collected three more events that are considered strong candidates for a pair instability supernova. In general they are probably very rare, only one in 100,000 supernovae are like this, and they seem to require a star of at least 140 solar masses and maybe even 200 or more. But they function as huge factories for creating elements, and they generate the most energetic explosions known to science - perhaps they should be called "hypernovas."
Perhaps the most spectacular aspect of this new type of supernova is that it gives us a glimpse into the early universe. The very first stars to ignite, about 100 million years after the Big Bang, had to be at least 100 times the mass of the Sun, and perhaps even 1,000 times. Some of these whales apparently exploded through a mechanism of pair instability. It is therefore possible that the distant cousins of some of today's supernovae were the first explosions that seeded the universe with heavier elements, and thus actually shaped the stars and planets that followed them, including our Sun and Earth.
Not only do our observations suggest a novel way for stars to explode, they also mean that, contrary to previous views, supergiant stars are likely scattered throughout the modern universe. Growth to such massive dimensions was possible for the primordial stars only in an environment consisting almost exclusively of hydrogen and helium. "Pollution" by nuclear fusion products restrained the growth of stars through the absorption of matter: in the presence of heavier elements, the stars collapse faster and therefore ignite earlier, blowing away any remaining gas in their surroundings before they can increase their mass too much. But there is now no doubt that the heavy elements inhibit stellar growth less than astrophysicists thought.
The supernova survey that Nugget and I started planning in 2007 is alive and kicking today: it is called the Palomar Transient Factory (PTF). As part of this project, we are looking for more examples of pair instability explosions; In fact, the project has allowed us to find some of our latest candidates for such an event, which look very similar to SN 2007bi. With the accumulation of data, we understand more deeply these explosions and how they contribute to the creation of the heavy elements in the universe. Future instruments, such as NASA's Next Generation Observatory, the James and Webb Space Telescope, will likely be able to detect very distant pair instability bursts. Maybe one day they will reveal the dying explosions of the first stars ever to form in our universe.
_________________________________________________________________________________________________________________________________________________________________
About the author
Avishai Gal-Yam completed his PhD in astrophysics at Tel Aviv University in 2004 and was a research fellow in the Hubble Postdoctoral Program at the California Institute of Technology (Caltech). He is currently a senior scientist at the Weizmann Institute of Science in Rehovot.
And more on the subject
How to Blow Up a Star. Wolfgang Hillebrandt, Hans-Thomas Janka and Ewald Müller in Scientific American magazine, Vol. 295, no. 4, pages 42-49; October 2006.
A Massive Hypergiant Star as the Progenitor of the Supernova SN 2005gl. A. Gal-Yam and DC Leonard in Nature, Vol. 458, pages 865-867; April 16, 2009.
Supernova 2007bi as a Pair-Instability Explosion. A. Gal-Yam et al. in Nature, Vol. 462, pages 624-627; December 3, 2009.
Scientific American Online
To see an interactive animation of stellar explosions, visit
ScientificAmerican.com/jun2012/gal-yam
3 תגובות
Fascinating, simply fascinating...
Avishai Hello and thank you for the interesting article.
According to what I understand, today it is known that after a supernova, a neutron star, a black hole, or in the case described in the article, nothing remains from the star.
I wanted to ask if we have observations that can tell what happens to the satellites of the star that goes supernova (planets or smaller stars) after the event is over?
Thanks
flint
Video about all kinds of sizes of stars in the universe:
http://www.youtube.com/watch?v=HEheh1BH34Q