Neutrino detectors usually reside in the earth's crust and weigh tons. A group of researchers announced in an article in SCIENCE that they were able to detect a neutrino effect on matter in a tiny desktop device
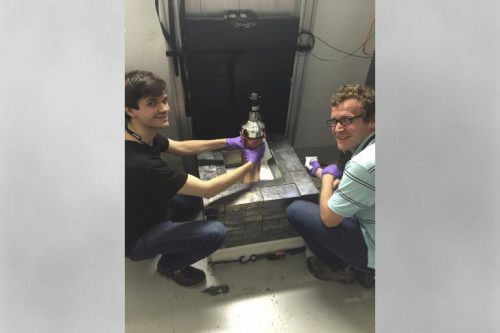
Neutrinos are one of the basic particles that make up the universe. However, compared to other types of particles, they have a very small mass, they have no electric charge and they interact with other particles using the weakest forces in nature - the weak nuclear force and gravity. Because of this, detecting evidence of their light collisions with other particles is a difficult operation that requires heavy instruments located deep in the earth to protect them from interference.
However, eighty researchers from all over the world who used the Spallation Neutron Source (SNS) research facility located at Oak Ridge National Laboratories succeeded in discovering neutrinos using a completely different method. As part of the COHERENT Experiment, these findings confirm a prediction made 43 years ago and open up new research directions for neutrino particles.
In an article published in the journal Science, signed by 80 scientists from 19 institutions in four countries (USA, Canada, Spain and Russia) that they managed to discover encounters of neutrino particles with other particles using a detector the size of a drink bottle, for over a year.
According to Jason Newbey, physicist at Oak Ridge and COHERENT's technical coordinator, says: The unique physical experiment facility at Oak Ridge National Laboratory was the first to measure consistent (coherent) scattering between low-energy neutrinos and atomic nuclei.
The standard model of particle physics holds that neutrinos are leptons, particles that collide very weakly with other particles. They are created as a result of radioactive decay - the nuclear reaction that drives the stars, as well as in supernovae. The big bang model of cosmology also predicts that neutrinos will be the most abundant particles in the universe because they are a byproduct of the creation of the universe.
As such their research was focused on theoretical physics and cosmology. In previous studies, neutrino particles were discovered by using tons of materials and then detecting changes in the position of the particles that are created as a result of a neutrino hitting them.
Examples of this are the Super-Kamiokande Observatory in Japan - an underground facility containing 50 tons of highly purified water. Another facility is SNOLAB – Sudbury Neutrino Observatory located in an abandoned mine near Sudbury, Ontario, Canada. This detector is based on heavy water. And of course, perhaps one of the most famous is IceCube - the world's largest neutrino detector, located at the Amendsen-Scott South Pole Station in Antarctica - relies on the Antarctic ice to detect neutrino interactions. In all cases, very isolated facilities that rely on very expensive equipment.
The COHERENT experiment, on the other hand, is much smaller and more economical in comparison, it weighs only 14.5 kg and takes up much less volume. The experiment was built to take advantage of the SNS accelerator's existing system, which produces the neutron rods designed to break apart mercury atoms by bombarding them with protons. This process creates huge amounts of neutrons that are used in various scientific experiments. However, the process also creates a significant amount of neutrinos as a byproduct. To take advantage of this, CoHERENT team members began developing a neutrino experiment known as "Neutrino Alley", located in a basement corridor only 20 meters from the mercury tank with thick concrete walls separating them to provide the system with natural shielding.
The corridor is also equipped with large water tanks to block additional neutrinos, such as those coming from cosmic radiation. But unlike other experiments, the COHERENT detector looks for signs of neutrinos colliding with other atomic nuclei. To do this, the team adapted the corridor with the detectors and added a detector based on cesium iodide crystals and also containing sodium aimed at amplifying the light signals caused by neutrino interactions.
Thanks to their experiment and the sophistication of the SNS, the researchers were able to determine that neutrinos are capable of coupling to quarks by exchanging neutral Z bosons. This process, known as Coherent Elastic Neutrino-Nucleus Scattering (CEvNS), was predicted back in 1973 by Prof. Daniel Friedman of MIT but until now, no experiment or research team has been able to confirm it.
Prof. Mark Karliner, professor of theoretical physics at the School of Physics and Astronomy at Tel Aviv University, explains in an interview to the Scientist website the importance of the find:
"Usually the neutrino sees each proton or neutron inside the nucleus individually, so the most natural thing was to think that the chance of a neutrino particle to interact with a nucleus is the sum of its chances of colliding with all the protons and neutrons inside the nucleus. Let's take for example an oxygen atom that has eight neutrons and eight protons, the chance of hitting the nucleus is the sum of the chances of hitting each of its components separately, that is 16 times."
"But the interaction between the neutrino particles that cross the atom and the nucleus of that atom is a quantum process and in quantum mechanics a particle like a neutrino sometimes behaves like a particle and sometimes like a wave. What determines whether it behaves more like a particle or more like light like a wave is the quantum equivalent of a wavelength. The greater the neutrino's energy, the more it behaves like a particle and less like a wave."
"In this experiment, they used neutrinos whose energy is relatively low, so their wavelength is relatively long, and then one wavelength of the neutrinos is of the same order of magnitude as the entire nucleus, and then the "neutrino" sees the entire nucleus as one piece. Then the rule of quantum mechanics that the chance of interaction behaves like the square of the overlap between the wave and the particles comes into play. Again for the example of oxygen, the chance is not 16 times but 16 times squared ie 256 times., meaning the chance of a neutrino particle colliding with a nucleus increases dramatically. Since the chance increases dramatically you don't need such a large detector. You can be satisfied with a small detector the size of a thermos. "
However, this process has a limit. If we take a neutrino with a very small energy (and equivalent to a very large wavelength) its collisions with the protons and neutrons would be so subtle that we would not be able to detect them. There is some gold value that is large enough on the one hand to make these nuclei move and can be detected inside the detector and on the other hand the energy value is small enough that the wavelength is such that they see the entire nucleus as one piece.
Since the atomic weight of cesium and iodine is over 50 (iodine 53, cesium 55), it is possible to be satisfied with a detector that is fifty times smaller than a detector that looks for interactions that behave linearly to the number of protons and neutrons.
There is no new physics in this story that was not known before but it is a beautiful demonstration of a somewhat counterintuitive rule of quantum mechanics. This discovery can have practical consequences - for example, the discovery of illegal activities in the nuclear field or leaks from nuclear power plants before they spread (neutrinos are a side effect of atomic reactions AB)
According to Prof. Karliner, this effect is very important in astrophysics. When a star explodes as a supernova, a tremendous flow of neutrinos comes out of its core because there is the energy that allows this, and therefore the interaction of these neutrinos with the material of the star that is in the mantle is much greater than it could be if it were neutrinos with a shorter wavelength. Each individual neutrino hardly moves to this nucleus, but the flux of neutrinos inside the supernova is so great that they explode the star's envelope, like Gulliver's stories that a lot of dwarves can overcome a giant. In conclusion, Prof. Karliner says: This is a beautiful and impressive demonstration of a phenomenon that is at the basis of quantum mechanics. Until today, neutrinos could only be seen in huge detectors that weigh tons, and this time they managed to measure them using a portable detector.
As mentioned, Prof. Friedman from MIT proposed the idea already over 40 years ago, but in order to do the experiment, Barka was required - in thinking of a way to build the facility in such a way that its sensitivity would make it possible to detect a tiny effect.
More of the topic in Hayadan:
4 תגובות
Father, this is a very fascinating subject, but I am not clear about the purpose. I guess they didn't read the article enough and wanted to increase exposure.
To me, it's a shame that such a fascinating subject is lost, but after "the people" have had their say, I see no point in it. Especially those who are looking for the new topics are disappointed
I did not return. I brought her back up
What will we be? Recycling articles again? This cycle is not always beneficial to the environment...
The reduction of neutrino detectors can certainly lead to far-reaching new discoveries in physics, for example if such detectors are launched into space, or perhaps even with the help of such detectors they build neutrino telescopes that will detect from which direction it comes and what its sources are and discover sources of such radiation in space.