A small test kit can diagnose infections within 20 minutes, thus shortening the waiting time for results by whole days and saving a life/year or Kelly
The article was published with the approval of Scientific American Israel and the Ort Israel network
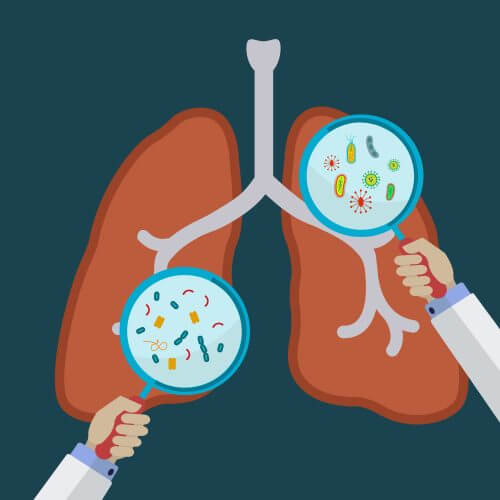
We routinely measure vital signs of health like body temperature or blood pressure, but we still don't have a way
Quick to accurately identify the cause of most infections. The patients pay a high price for our inability to recognize harmful viruses and bacteria. During the waiting days usually needed for doctors to identify bacteria and viruses, the diseases spread in the body and treatment becomes more difficult, and the most vulnerable patients - newborns, adults and those with weak immune systems - may die.
These delays occur even in clinics equipped with all the advantages of high-tech medicine, not least in small clinics in Africa where receiving the results can take many days. During this time, malaria patients may receive the wrong typhoid treatment and Ebola patients may be left without special supervision, instead of being sent to isolation.
The tests are so slow because the molecular fingerprints of any particular infection are hidden in the human body among the abundance of proteins and normal particles that hide them. A single blood sample has perhaps 1,000 molecular markers unique to a particular bacterium floating among trillions of molecules unrelated to the infection. It takes a long time for expert scientists, in specialized laboratories equipped with complicated and expensive equipment, to find a large enough group of target molecules to trigger the alarm signal.
We are now on the verge of a marked improvement in the situation. Instead of wasting time and risking lives in transferring the samples from the patients to the diagnostic laboratories, we can discover the molecules that characterize the disease and identify them already in the doctor's clinic, after waiting about 20 minutes. This can be done with the help of tiny nano-sensors, billionths of a meter in diameter, contained in a small plastic container. All you have to do is put a drop of blood in the container and get the results. The nano sensors respond quickly to low levels of bacterial DNA, in part because the sensors and the DNA are roughly the same size.
And size does matter. A small wave will not be felt in a battleship, but it will shake a rowboat fairly well: it will splash water over the sides of the boat and startle the rowers. So are our nano sensors: they react to their environment, the liquid in the blood sample, in a way that sensors larger than them are not capable of, and this happens very quickly.
My colleagues and I are excited to know that our systems will be tested in clinics during 2016. And our system is just one of several promising diagnostic approaches developed by other researchers who also use nanoscale reactions. In the last decade scientists have included methods to design materials, often atom by atom. Laboratories around the world use these precise control methods to design and build devices that respond specifically to predefined conditions. The response time of these devices is very short compared to the previous large devices. We're all still very cautious because we've already seen successful prototypes fail in the real world. But we also hope that such methods will eventually help us bring a cure when it is needed.
catch disease
My research group entered the field about ten years ago. We have watched in awe as people with diabetes use simple glucose sensors that can be comfortably held in the hand. The glucose molecules actually close an electrical circuit in the device and create a current by losing some electrons. A stronger current indicates more glucose in the blood. We wondered if we could use the same approach to measure certain DNA and RNA sequences from bacteria or viruses that serve as unique markers of infection.
In order to achieve the goal, we had to find a way to attract and capture some DNA molecules from the causative agents of the disease that may be found in a blood sample taken from a patient. For fishing we needed bait. One of the nice features of each piece of DNA is its tendency to adhere selectively and tightly to another sequence of DNA that we can design and prepare ourselves. So for example we can create a sequence to fish out a strain of Staphylococcus bacteria. This is a highly unique bait. We connected the bait molecule to a sensor, a millimeter wide gold wire, designed to transmit an electric current only when the bacterial DNA is captured. (Gold works well because it is a good conductor of electricity.)
But since DNA by itself does not release enough electrons to produce an electric current strong enough to measure with the gold wire, we needed an amplifier. For this we added particles of the metal ruthenium to our sample. These metal particles have a positive electrical charge so they are attracted to the DNA which has a negative charge. And when a DNA molecule binds to our sensor, the metal particles join it. The metal-DNA coupling easily attracts electrons from the gold wire, and thus an electric current is created with a power that can be detected. Different bait molecules on the surface of the sensor allow us to locate DNA from different bacteria.
The bad news came when in situations similar to real life this method did not work. It worked well when we introduced a large amount of bacterial DNA into our samples: trillions of molecules. But then we tried the method with DNA levels close to the levels usually found in blood samples drawn by a doctor with a needle. Such samples typically contain about 1,000 target molecules or less. We tried to skew the results in our favor and used samples with a million target molecules, but even then we failed to get a detectable signal. We were very far from our destination.
Diagnosis - small device, huge response
For a year we tested all the variables in our system trying to understand why we could not detect a smaller number of molecules. It was frustrating: no trick we could think of made the method more sensitive. Some students in the group gave up and asked to switch to other subjects. I too began to wonder if my research group would survive.
Luckily for us, the hand of chance played on our side. One day in 2004 we held a discussion on another study, not related to this topic but also in which gold wires were used, albeit on a much smaller scale. The diameter of these nanowires was only 10 nanometers (10 billionths of a meter), a diameter that could only hold five DNA molecules. So just for fun, and because nothing else worked, we replaced the gold wires we've been using so far, which are a millimeter in diameter, with the nano wires and did some quick and "dirty" experiments just to see if anything would happen.
And something did happen. Rahela Gaspark, who was then a postdoctoral student in the group, came running to my office clutching a piece of paper with the results of the first experiment. The nanowires increased the sensitivity a million times. At first we wanted to go out to celebrate, but on second thought we realized that we needed to repeat the experiments and headed back to the lab. We wanted to be sure that what we saw was real. And indeed it was. At that moment we knew we had a way to locate those 1,000 molecules that would allow us to diagnose diseases.
Why did nanowires allow us to sense much lower concentrations of DNA? This happens because the size of the wires has a significant effect on their shape. When moving to a nanometer scale, small and pointed bumps appear on the wires that are not found on the surface of the larger wires whose volume gives them a flat and smooth surface area. With one bait molecule attached to one side of a bump and another to the other side, there is a space around them that is larger than a marble around the molecules crowded onto the flat, larger wire surface. A liquid can move more easily in this space carrying the target molecules with it. The bait molecules and the target molecules therefore have a higher chance of meeting and binding with each other.
These sensors were good, but the students in the group could only handcraft ten of them a day. For medical use we will need thousands of such sensors. We therefore did what many scientists and engineers before us did, when they wanted to produce many electrical devices, and we turned to silicon.
Fig. Curves on a nanometer scale
Electrodes can be attached to chips made of silicon and mass produced. We wanted to take the nanometer bumps that characterized our nanowires, those pointed bumps that enhanced sensitivity, and replicate them on such a chip. After about six months we found a good way to do this using electro-chemical plating. We could start by placing larger, micrometer-scale structures on top of the silicon and then use the chemical coating and put thinner layers of gold on top of them. Instead of growing nano-wires, we learned that it is faster and easier to create a kind of gold dome with many spikes. By fixing bait molecules around the spikes we mimicked the separation created by the bumps in the original wires. The key was timing. If the coating process lasted a little longer than desired, the structures would swell to a size that would not allow use. But if you stop the process after a short period of time, the structures reach a nanometer scale and their growth stops.
gable
In the years since initial development, we have shown that we can use these detectors to analyze markers of infectious disease-causing bacteria and that we can determine the presence or absence of such a pathogen within 20 minutes. This period of time is very important because in order for the diagnosis at the clinic to be successful, it is desirable that the answer to the test arrive within the typical time of a visit to a doctor. Another important aspect of our approach is what we call "multitasking": the ability to search for many pathogens at the same time. We were able to place many gold domes on the surface of our chips and attach to each dome a different type of bait molecule. This way we can diagnose many types of pathogens with a drop of a single blood sample on the chip. Most other approaches can only test one type of pathogen DNA at a time. One of our more ambitious studies was to test 20 different bacteria at once along with five common DNA markers for antibiotic resistance. We were able to diagnose them with 99% accuracy.
In an attempt to market the technology to doctors' clinics, we established a commercial company, Xagenic. The company, in which I am in charge of the technology, built a plastic device around our chip and integrated everything necessary for diagnosis. These devices will be tested in clinical trials that will begin in 2016 and will test their accuracy in diagnosing two sexually transmitted diseases, chlamydia and gonorrhea. Doctors and their patients in 20 clinics will participate in the tests. If the first trial is successful, we intend to submit the results to the US Food and Drug Administration (FDA) and seek approval to launch a commercial product.
We compete with other promising nanotechnologies, and the competition is fierce. Some of these tests focus on certain types of cancer and reach a new level of precision. Chad A. Mirkin's group at Northwestern University, for example, has developed gold nanoparticles that react with the DNA of cancer cells even before the dangerous cells form tumors. David Walt of Tufts University has developed a system that counts molecules used as a marker for diseases and determines how many such molecules are present in a patient's blood, information that may be essential for cancer diagnosis and monitoring. But all these approaches were designed for use in diagnostic laboratories and not necessarily in doctors' offices.
But there are also other methods that focus on on-the-spot diagnosis, and they are making their way into everyday medicine. Rostem Ismagilov's group at the California Institute of Technology developed a wireless device called the SlipChip, which enables DNA detection without needing to be connected to an electrical outlet. In early 2015, Samuel Seah and his colleagues from Columbia University reported in the journal Science Translational Medicine about a tiny blood sample connected to a cell phone that detects HIV by detecting antibodies in the blood.
I believe that one or more of these technologies, or a completely different technology that we still don't know about, will eventually work at a level good enough for everyday medical use. Then, chemical reactions carried out in a device that is a millionth or a billionth of a meter in size will improve the health of patients beyond any scale.
4 תגובות
during the exile.
Correction 1 milliwatt per square meter. 1,000 times less. It's still quite a bit of radiation.
Besides that we have caused considerable damage to our environment which causes diseases in ways that did not exist before. It was recently learned that rice and other grains, especially wild rice because of the husk, increase the chance of eye cancer by 49%, not because of the rice, but because of its ability to store arsenic and the factories that also exist in Asia that emit arsenic. This is a basic food type. Similarly, we live in a radiation bath of at least 4 milligauss at a frequency of 50-60 Hz and of about 1 watt per square meter at 2 gigahertz. When it was found that some families in Sardinia reach the age of 100 on average and some even 115. There is also genetics here, but on this island there are fewer power lines, certainly high voltage, fewer cellular antennas, and there are up to two workers and that's it. Bottom line, if they allowed us to live healthy lives, less technology would be needed to cure them.
We know and know the Prophet Muhammad, who is very poor of all people, and we see Zeresh and his enemies, the evil snakes, who for many years fight and beat and oppress him with terrible, terrible cruelty, do not give him contact with his children, and spread a bad name and lies about him and fight to destroy his life, angels of destruction, who do not know Only to take control, and they don't care at what cost, that's why we ask the public to understand his situation and help and support him. The people of Israel will praise the three signs in the old days, merciful and reciprocating kindness, strengthen him and save him from his enemies and those who seek his harm.