The institute's scientists succeeded in merging two low-energy photons into one high-energy particle
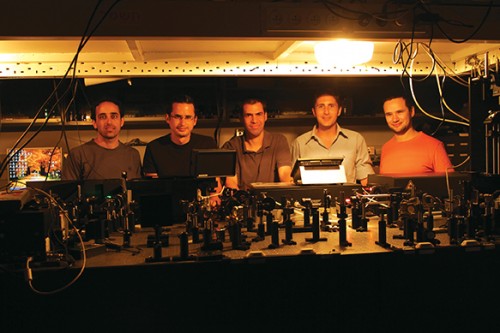
Sunlight can provide all of humanity's energy needs, but solar energy still makes up a tiny fraction of the total global energy market. One significant obstacle in this area is inefficiency. For example, when solar cells convert sunlight into electricity, most of the energy is lost: about a quarter of the energy is wasted because the cells can absorb light particles, called photons, only above a certain threshold of energy. All the photons that are of low energy are wasted, which reduces the efficiency of the cell and increases the cost of energy production.
One possible solution is to turn two low-energy photons into one high-energy particle, which will be absorbed by the solar cell, but this process is a huge challenge. Just as it is easier to break a vase into pieces than to put it back together, so it is easier to break a particle than to fuse two particles into one. It is particularly difficult to obtain as a result of this process photons of a certain color, that is, of a certain energy level. Now Weizmann Institute scientists have developed an innovative method to overcome the difficulty. The research, which was published in the journal Nature Nanotechnology, was carried out by Prof. Dan Oron and research students Zvika Deutsch and Lior Naman, from the Department of Physics of Complex Systems.
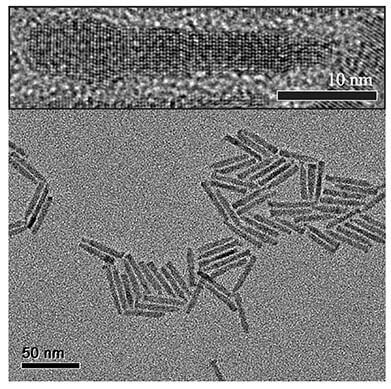
With the help of chemical processes based on solutions, the scientists built nanocrystals in the form of sticks that are about 50 nanometers (billionths of a meter) long. To illustrate, in the area occupied by the dot at the end of this sentence, about a billion such crystals can be inserted. At one end of the nanotube there is an electron that absorbs photons one after the other. In the first stage the electron is excited by one photon, and then it is pushed to a higher energy level by the next photon. Thus it becomes a high-energy electron, which is transferred to the other end of the beam, where it emits a photon with a higher energy than any of the absorbed photons.
With this method, the scientists managed to turn two red photons, with a relatively low energy, into one green photon with a higher energy. In fact, the system can be tuned to almost any color: it allows great flexibility, because you can control the properties of the nanomaclons by changing their diameter.
To help nanocrystals move from the laboratory to industry, the scientists are now trying to increase the efficiency of the system, and achieve better control over the colors of the photons. In the future, it may be possible to use these types of crystals in combination with more common materials, such as silicon, the material from which most commercial solar cells are made. Silicon does not absorb photons from the infrared level and below, and it may be possible to capture these photons with the help of the nanocrystals.
Focus on time
When it comes to brain research, the term "enlightenment" may have several meanings. Research that sheds new light on the activity of the brain can be carried out using "illuminating methods" - those that involve stimulating the nerve cells in the brain, the neurons, with a light beam. For example, a beam of light can be directed at individual neurons to find out which other neurons they communicate with. In the end, the scientists can discover in this way complete networks of neurons responsible for all our functions, from memories and emotions to movements and behaviors.
Although, it is possible to study these networks using electrodes, but a light beam is less invasive, and it is easier to move it from place to place. The only problem is that when the beam is aimed at an area deep within the brain, it illuminates the entire area, and not just the target neuron.
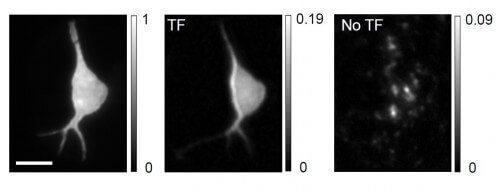
In a study carried out in collaboration with scientists from France, Prof. Dan Oron and members of his group found a solution to this problem. As reported in the journal Nature Photonics, they were able to stimulate neurons deep within the brain tissue - a layer of the mouse brain that exceeds 200 microns in thickness - using short impulses (pulses) of laser. Prof. Oron's group included the research student Ben Leshem and the post-doctoral researcher Dr. Osip Schwartz from the Department of Physics of Complex Systems. They collaborated with Dr. Valentina Emiliani's group from Paris Descartes University - Dr. Irini Papagiacomo and Orleans Bega - and with her colleagues, Dr. Brandon Stell and Dr. Jonathan Bradley.
The research was based on a method called "temporal focusing", previously developed at the Weizmann Institute of Science. As can be learned from its name, it is about controlling the temporal focus of the laser beam, not spatial focus. With this method, the sample is illuminated with long pulses of light, which are shortened when the beam reaches the desired layer, where it causes the desired illumination. As a result, only the target neuron, which has been genetically engineered to respond to short pulses, but not to long pulses, is excited. Furthermore, with this method, the neuron is illuminated uniformly, and its borders are sharply defined. The sharpness is possible because the focus in time dramatically reduces the scattering of light within the brain tissue. Some of the photons are indeed scattered, but they do not interfere with the excitation because they do not reach the brain tissue at the "right" time, that is, during the illumination of the target neuron. The method enables extraordinary precision thanks to the short length of the pulses, which last only about one hundred femtoseconds (each femtosecond is a millionth of a billionth of a second).
Using this method, it will be possible to study neuronal networks by exciting individual neurons with short laser pulses.