Two experiments to measure the radius of the proton provided two completely different values. What's going on here?
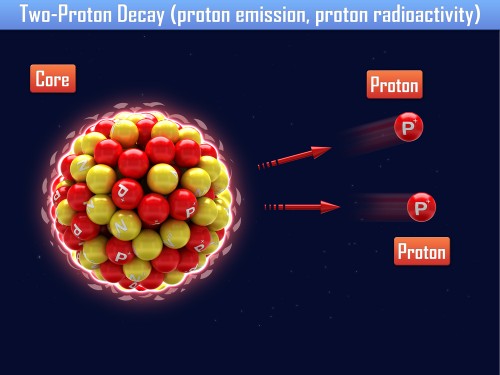
The assumption that we understand the proton is a forgivable mistake. After all, the proton is the main component of matter in the visible universe, the fuel that fuels the furnaces in the hearts of stars. The century-long studies of the proton, the positively charged particle that binds to a corresponding negatively charged electron to form a hydrogen atom, were the opening shots of the quantum mechanics revolution. Today, researchers accelerate protons to high energies and generate a flood of collisions between them to conjure up exotic particle phenomena such as the Higgs boson.
However, recent studies on the proton surprised us. The two of us (Bernauer and Pohl), together with our colleagues, have made the most accurate measurements of the proton radius to date with the help of two complementary experiments that approached the problem from two complementary perspectives. When we started this exercise, we imagined that our results would help add levels of precision to the known size of the proton. We were wrong. There is a huge gap between the results of the two measurements of the proton radius that we performed: more than five times the rate of the error range in each of the measurements. The probability that this all happened by chance is less than one in a million.
Obviously something is not right. Either we do not understand the proton correctly, or we do not understand the physics underlying the exact measurements. We reached into the depths of the universe and pulled out something unusual. And so we have a wonderful opportunity to learn something new.
The missing offset
Our story begins on the Italian island of San Cervolo, ten minutes by speedboat from St. Mark's Square in Venice. Until the end of the 70s of the 20th century, there was a mental hospital on the island. Thirty years after the hospital closed, several dozen physicists began holding conferences on the island to discuss more and more rigorous ways to examine the most understood theory in the entire world of physics, and perhaps even in all of science: quantum electrodynamics, known for short as QED.
The roots of QED are planted somewhere in 1928, when the physicist P. A. M. Dirac combined quantum mechanics and special relativity and created from them what is known today as the Dirac equation. It is the best theory we have for electricity and magnetism because it provides a complete explanation of the way light and matter interact with each other. For one of many examples, QED explains the structure of atoms using the laws of physics and the values of fundamental constants such as the electron mass. For this reason physicists use simple atoms like hydrogen to test QED. They can predict the results of experiments with a margin of error of 0.0000000001%. And the experiments correspond to this level of accuracy.
We met each other for the first time in San Cervelo. We both started making measurements of the proton to refine our knowledge of QED. Bernauer's experiment was designed to study the internal structure of the proton using an improved version of a method that yielded the most precise measurements up to that time.
Pohl's group relied on a new approach. She examined elusive shifts in the energy levels of an exotic type of electronless hydrogen, shifts on which the size of the proton has a decisive effect. These shifts were first identified in ordinary hydrogen by the late physicist Willis Y. Lamb Jr. as early as 1947. Although the physicists call the phenomenon by one name, "hist lamb," they realized that two distinct causes play a role in its creation.
The first contribution to hist lamb comes from particles known as virtual particles, ghosts that pop up inside the atom and quickly disappear again. Scientists can use QED to calculate with remarkable precision how these virtual particles affect atomic energy levels. But in recent years there has been uncertainty about the second reason for the Lamb shift, which limits scientists' predictive powers. This second reason has to do with the radius of the proton and the strange quantum nature of the electron.
In quantum mechanics, the electron takes the form of a cloud-like wave function that is spread over the entire volume of the atom. The wave function (or more precisely, the squared wave function) describes the probability of finding the electron at a given location, and it can only appear in certain discrete forms, which we call atomic states.
The wave function of some of the atomic states, known for historical reasons as "S states", takes on a maximum value in the atomic nucleus. That is the probability of finding the electron בתוך The proton itself is not zero, and it increases with the proton radius. When the electron is inside the proton, it does not "feel" the electric charge of the proton as much, and this reduces the total binding force between the proton and the electron.
This reduction of the binding force changes the lambda shift in the lowest energy state, labeled "1S state", by 0.02%. The magnitude of the shift may seem negligible, but the energy difference between the ground state, 1S, and the first excited state, labeled 2S, is measured with an amazing precision of 14 digits after the dot. Therefore, even a tiny effect of the proton radius must be taken into account if one wants to compare the QED theory with precise experimental measurements.
Pohl's group has been trying for eight years to accurately determine the size of the proton. But in the days of the first conference in San Cervelo, her experiment seemed to be malfunctioning, and no one could figure out what had gone wrong.
In the meantime, Bernauer's team was to begin additional research on the proton radius. His approach was not to rely on energy levels of hydrogen, but to use electron scattering from a hydrogen target to infer the exact size of protons.
Shooting towards the targets
Hydrogen gas is essentially a swarm of protons. If you shoot a beam of negatively charged electrons at him, some of them will be deflected by a positively charged proton and "scatter" away from the original direction of the beam. Furthermore, this scattering depends to a great extent on the internal structure of the proton. (Protons, unlike electrons, are made of more elementary elements.)
Let us take a closer look at the interactions between a proton and an electron in such scattering. When the electron scatters, it transfers part of its momentum to the proton. In QED, the physicists describe this interaction as the exchange of a virtual photon between the electron and the proton. If the electron scatters only a small amount, and is slightly repelled, it transfers only a small fraction of its momentum to the proton. If it scatters at a rate close to 180 degrees, we describe it as a head-on collision between the electron and the proton and then it has transferred a decent amount of momentum to the proton. In QED, high momentum means that the virtual photon has a smaller wavelength.
As in a light microscope, if we are interested in seeing the smallest structures, we use the shortest possible waves. Bernauer's work was, among other things, to use short waves to study the charge distribution inside the proton.
However, when Bernauer went to a conference in San Cervelo, the scientists he met there asked him to expand his experiment. Short waves are indeed suitable for observing the structures inside the proton, but if you are interested in examining the proton as a whole, you must use longer waves. In fact, if you are interested in measuring the proton as a whole (and its radius anyway), you have to use an infinite wavelength, which allows the photon to "see" the entire proton. This is the limit where no scattering occurs.
Technically, this is of course impossible, the electrons must deviate at least by a small amount for a measurement to be possible. Therefore, Bernauer's group measured the lowest momentum transfer allowed by the experimental setup and then extrapolated the results to zero.
His efforts succeeded in almost halving the gap between the lowest momentum transfer measured up to that time and zero, and therefore the extraction was much more reliable than the extraction in previous experiments. In the end, the number of measurements conducted in the experiment was about twice as large as the number of all previous measurements combined. After conducting the experiment in 2006 and 2007, it took Bernauer three years to analyze all the data. Work that earned him a Ph.D. He found that the radius of the proton is 0.879 femtometre (10-15 meter) approximately, about ten billionths of the size of a fog drop, a size that matched the previous measurements with exact precision.
strange hydrogen
Meanwhile, Pohl and his team members continued to struggle. In their experiment, they replaced the electron found in a regular hydrogen atom with its heavier cousin - a muon. Muons are almost completely identical to electrons, and the only difference between them is that the mass of the muon is almost 200 times greater than the mass of the electron. This difference causes the muon in muonic hydrogen to be approximately 200 times closer to the proton than the electron in a normal hydrogen atom.
If the muon is 200 times closer to the proton, then it should spend much longer בתוך the proton. (Indeed the probability of this has increased 200 times3 or 8 million times). Thus the lambda shift in such an atom varies by 2%, a relatively huge rate, which should be able to be easily identified.
Members of Pohl's team fired ions produced at an accelerator at the Paul Scherer Institute (PSI) in Switzerland into a tank filled with hydrogen gas. Occasionally a muon would exchange an electron with an atom, breaking the hydrogen molecule and creating a muon hydrogen atom in a highly excited state. Within a few nanoseconds the ionized hydrogen would drop to lower energy levels. In this experiment, only hydrogen atoms were used that eventually reached the first excited energy state (2S state).
Each time a muon entered the hydrogen tank, it sent a signal that activated a laser system, which approximately a microsecond later fired a laser pulse. If the amount of laser energy, which can be measured by its wavelength, was just the right amount, it would jump the atom from the 2S state to the higher energy 2P state. The spatial shape of the 2P state does not allow the muon to be inside the proton [see box on the next page], so if we measure the energy difference between the 2S state and the 2P state, we can deduce how much time the muon spent inside the proton, and in any case also the radius of the proton.
But here was the minefield: we had to tune the laser to match exactly the right amount of energy. The atom will jump to the higher level only if there is a perfect match between the energy of the laser and the energy difference between the 2S state and the 2P state. If the wavelength exceeds a little nothing happens. So how could we know if the atoms had made the jump? Every atom that is kicked up to the 2P state releases in a short time a photon in the low energy range of X-rays. If we find such photons, we know that the laser is at the right energy.
This sounds simple on paper, but these experiments are notorious for the hardships they pile on the experimenters. Similar experiments were first proposed back in the 60s, when QED was still a relatively new theory, as a test to see how accurate it was. But the experiment was more difficult to perform than complementary experiments on hydrogen and other electron atoms, so interest waned until the 20s, when these tests became limited by the uncertainty of the proton radius.
Pohl's group proposed the Lamb shift measurement of ionized hydrogen to the directors of PSI in 1997. The institute approved the project in early 1999, and we spent three years building a laser system, a beam of low-energy muons and low-energy X-ray detectors.
At the end of the construction of the experimental setup at PSI in 2002, we had to deal with some technical issues. After we were able to settle them, we only had a few hours left to actually shoot laser beams at ionized hydrogen before our allotted time to use the accelerator ran out. Some of us were very disappointed because we really believed we would be able to find the 2S-2P shift in "one shot". But senior physicists were more realistic about the prospects of the first run of "developing the machine." They were happy that everything worked properly and that only a few minor technical problems surfaced. These problems, they believed, would be able to be fixed before the start of the "real run", which was to take place in 2003 and in which we believed that we would surely see the sign of Hist Lamb.
Then, after many months of preparation and three weeks of successful data collection, we discovered nothing. Not even a single letter difference. Although the laser scanned the entire range of wavelengths that matched the known experimental values for the proton radius. Nothing.
We assumed the obvious: obviously something in our experimental setup is not right. The conclusion was that we had to improve the laser system. We began an extensive redesign, which was completed in late 2006. We collected data for another three weeks in 2007 and again saw nothing. Fortunately, we were given one last chance in the second half of 2009. It took us several months to get the complex facility up and running. And again, after a week of collecting excellent data, we found no sign of a signal.
We were signed up for just one more week of observations. We were afraid that if they failed someone in the management would decide that this task was too big for us. The experiment, which has been going on for ten years, will be declared a failure and will be closed for good.
And then we finally started to wonder if something deeper was going on here. What if we're looking for the proton radius in the wrong place? We decided to expand the search range. The group members jointly decided to look for a larger proton radius. But one evening, late at night, Pohl's colleague, Aldo Antonini, entered the control room and said he had a feeling it was worth looking for a proton smaller. Under the constraints of pressing time, Pohl and Antoineni reoriented the search to look for a proton radius smaller than anyone had ever imagined. In a very short time we discovered a hint of a signal. But the very next day, the accelerator was closed for maintenance that was scheduled in advance and lasted four days. We had to wait.
Then, on the evening of July 4, 2009, 12 years after the start of the operation, an unequivocal signal appeared, telling us that the proton measured in the muonic hydrogen was considerably smaller than everyone had thought until then. The group spent a few more weeks in additional measurements and calibrations, and a few more months in analyzing the data. The final result, which we have since confirmed with additional measurements, is a proton charge with a radius of 0.8409 femtometres, within an error range of 0.0004 femtometres. This number is ten times more accurate than all previous measurement results, but it differs from them by 4% - a huge discrepancy!
In 2010, the two groups reported their results at a conference on exact physics of simple atoms held in the village of Auch in the French Alps. Pohl first presented the results of the muonic hydrogen measurements to the scientific community. That same day in the afternoon data from Bernauer's experiment was delivered. Pohl and his colleagues expected Bernauer's analysis to back up the new, smaller result. But to their surprise the results were almost identical to the old radius: 0.877 femtometre.
new ideas
This contradiction caused enormous excitement in the community. Contradictions are useful because they stimulate new thoughts, which lead to new ideas and a better understanding of nature.
At first, most people thought that there must be a simple mistake here. Perhaps something went wrong during the experiments, and perhaps a mistake was made in the theoretical calculations required to extract the radius. A little after the conference unrelated researchers came and showered us with possible candidates for direct errors.
For example, prior to Pohl's experiment only three people had performed the complex calculations required to translate the experimental measurements of the laser's wavelength into the proton's radius. Many speculated that there were errors or omissions in these calculations. Therefore, many theorists repeated the calculations and expanded them, but did not find any error.
Others have tried to rethink how Bernauer deduced the radius from his dispersion data. Is it possible that we can reconcile the raw data with the smaller radius of the muonic hydrogen? Apparently, this solution was also rejected.
Each time a proposal was rejected, the contradiction became more and more jarring. Four years after the proton radius puzzle arose, physicists exhausted direct explanations such as errors in measurement or calculations. We began to dream of more exciting possibilities.
For example, do we really understand how the proton responds to the pull of the muon? The muon's electrostatic force distorts the proton's shape, similar to how the moon's gravity creates tides on Earth's surface. The bent proton slightly changes the 2S state in ionized hydrogen. Most people think we understand this phenomenon, but the proton is such a complex system that we may have missed something.
The most exciting possibility is that these measurements may hint at a new physics that goes beyond the so-called Standard Model of particle physics. It is possible that the universe contains an undiscovered particle that somehow causes muons to behave differently than electrons. Scientists are investigating this possibility, but they have found that it is not easy to build a model for a new particle without it also having observable effects that contradict the results of other experiments.
Physicists have yet another unsolved muon puzzle. Elementary particles such as muons and electrons have a "magnetic moment", a magnetic field that is very similar to a normal magnet. The muon's magnetic moment does not match the QED calculations, and this is telling. It is possible that a new physical phenomenon could explain both the proton radius measurements and the muon's unusual magnetic moment.
Several new experiments have been proposed to settle all these hypotheses once and for all. At least two scattering experiments are expected to improve the accuracy of the previous scattering experiments, one at the Thomas Jefferson National Accelerator Facility in Newport News, Virginia, and the other at the Mainz Microtron, at the Johannes Gutenberg University in Mainz, Germany, the university where Bernauer conducted his original experiment. These measurements will provide independent confirmation and test some of the proposed explanations.
Both Pohl's group and the team in Mainz want to measure the radius of deuterium, a nucleus with one proton and one neutron, to see if the difference shows up there as well. Pohl is also trying to measure ordinary electronic hydrogen with greater precision.
In addition, many physicists have pointed out that researchers have performed atomic measurements with both muons and electrons, but have performed scattering experiments only with electrons. The missing slot is a combination of muons and scattering. Bernauer is involved in a venture that will fill this void. Using one of the muon beams at PSI, the institute where Pohl's group performed their experiment, both electrons and muons will be scattered from protons in an experiment called the Muon-Proton Scattering Experiment (MUSE) to make a direct comparison. This experiment could test some of the most applicable explanations that have been proposed.
Time will tell if the radius puzzle will be remembered as a strange error or as a gateway that led to a deeper understanding of the universe. This riddle may well be the thread we must pull to uncover the next chapter in nature's book. And therefore we will pull on him.
__________________________________________________________________________________________________________________________________________________________________
in brief
A new experiment to measure the radius of the proton found it to be considerably smaller than expected.
The difference raises the possibility that the physicists do not understand something important about the proton itself or about the theory called quantum electrodynamics, which is so far the most understood scientific theory that has been put to the most rigorous tests.
With a little luck, this anomaly could lead to a thorough rewriting of the laws of physics.
About the authors
Jan K. Bernauer (Bernauer) is a postdoctoral student in nuclear physics at the Nuclear Sciences Laboratory at the Massachusetts Institute of Technology (MIT).
Randolph Pohl studies the laser spectroscopy of hydrogen and hydrogen-like exotic atoms at the Max Planck Institute for Quantum Optics in Gerching, Germany.
More on the subject
The Size of the Proton. Randolph Pohl et al. in Nature, Vol. 466, pages 213-216; July 8, 2010.
High-Precision Determination of the Electric and Magnetic Form Factors of the Proton. JC Bernauer et al. in Physical Review Letters, Vol. 105, no. 24, Article No. 242001; December 10, 2010.
Muonic Hydrogen and the Proton Radius Puzzle. Randolph Pohl et al. in Annual Review of Nuclear and Particle Science, Vol. 63, pages 175-204; October 2013.
The article was published with the permission of Scientific American Israel
One response
I didn't understand why they expected the size of the ionized hydrogen to be the same as the normal hydrogen.
The muon is closer to the atom and the whole distribution of the electric field is different. This surely affects the distribution of quark positions in the nucleus.