At the end of the 19th century, the famous German mathematician, Leopold Kroenker, stated that "God created the whole numbers and everything else is the work of human hands." He believed that whole numbers play a fundamental role in mathematics. This quote also speaks to the hearts of today's physicists, but in different tones. It links to the belief, which has become more and more accepted over the past decades, that nature, deep down, is discrete, that the building blocks of matter and of space-time can be counted
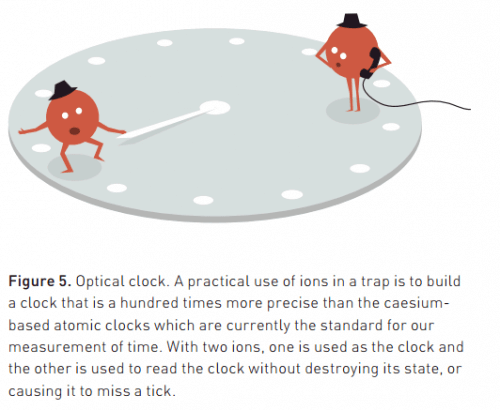
At the end of the 19th century, the famous German mathematician, Leopold Kroenker, stated that "God created the whole numbers and everything else is the work of human hands." He believed that whole numbers play a fundamental role in mathematics. This quote also speaks to the hearts of today's physicists, but in different tones. It links to the belief, which has become more and more accepted over the past decades, that nature, deep down, is discrete, that the building blocks of matter and space-time can be counted, one by one. The roots of the idea are already planted in the thought of the ancient Greek atomists, but it has more power today, in the digital age. Many physicists have come to think of the natural world as a supercomputer described by discrete bits of information, where the laws of physics are used as an algorithm, like the green digital rain that Neo sees at the end of the 1999 film The Matrix.
However, is this really the way the laws of nature work? Although this approach may seem to be at odds with the spirit of the times, I, and not only me, believe that reality is fundamentally analog and not digital. From this point of view, the world is a real continuum. Even if you look at it as closely as you want, you will not find building blocks that cannot be divided. Physical quantities are not integers but real numbers: continuous numbers, with an infinite number of digits after the decimal point. The well-known laws of physics, much to the disappointment of "Matrix" enthusiasts, have characteristics that no one knows how to create a computer simulation for, whatever the number of bytes in their memory. Recognition of this aspect of the laws of physics is essential if we wish to develop a unified and complete theory of physics.

An ancient riddle
The debate between digital and analog is one of the oldest debates in physics. The atomists, on the one hand, saw the world as discrete, and on the other hand, other Greek philosophers such as Aristotle thought of it as a continuum. At the time of Isaac Newton, who lived in the 17th and 18th centuries, the philosophers of nature were divided between particle theories (discrete) and wave theories (continuous). In Kronecker's time, atomists such as John Dalton, James Clerk Maxwell and Ludwig Boltzmann were already able to derive the laws of chemistry, thermodynamics and gases. But still many scientists remain skeptical.
Wilhelm Ostwald, winner of the 1909 Nobel Prize in Chemistry, argued that the laws of thermodynamics apply only to continuous quantities such as energy. Similarly, Maxwell's electromagnetic theory described electric fields and magnetic fields as continuous. Max Planck, who would later be one of the pioneers of quantum mechanics, concluded an influential paper in 1882 with the words: "Despite the great success that the atomic theory has enjoyed so far, it will eventually have to be abandoned in favor of the assumption of continuous matter."
One of the strongest arguments of the continuum camp was the apparent arbitrariness of non-continuity. For example: How many planets are there in the solar system? When I was in school, I was told there were nine. In 2006, astronomers officially removed Pluto from the group of planets, leaving only eight. And at the same time, they put together a BT league, of dwarf planets. If we include these, the number will reach 13. In short, if you want to honestly answer the question of how many planets there are in the solar system, we will have to say that the answer depends on how you count. The Kuiper Belt beyond Neptune's orbit contains objects ranging in size from a few microns to thousands of kilometers. You can name the planets only if you decide on a fairly arbitrary diagnosis that will determine what is a full planet, what is a dwarf planet, and what is just a block of rock or ice.
Eventually, quantum mechanics came along and changed the face of the debate about digitality and analogness. The definition of a planet may be arbitrary, but the definition of an atom or an elementary particle is not arbitrary at all. The integers attached to the chemical elements, which as we know today count the number of protons in the atom that make up the elements, are objective numbers. Whatever the future developments in physics, I'm happy to bet that we will never see an element with √500 protons that "sits" between titanium and vanadium. The integers in atomic physics do not plan to leave us.
Another example of this is the field of spectroscopy, that is, the study of the light emitted by matter and absorbed by it. A certain type of atom can only emit very specific colors of light, and the result is a distinct fingerprint for each and every atom. Unlike human fingerprints, the spectrum of each atom obeys stable mathematical laws. And these laws are governed by whole numbers. The first attempts to understand quantum mechanics, especially the attempts of the Danish physicist Niels Bohr, placed discrete quantities at its heart.
Integers emerge
But Bohr was not the final arbiter. Erwin Schrödinger developed an alternative approach to quantum theory in 1925, based on the idea of waves. The equation he formulated to describe how these waves develop contains only continuous quantities, without any whole numbers. And yet, when you solve the Schrödinger equation for a certain system, a little mathematical magic happens. Take for example the hydrogen atom: the electron orbits around the proton at very specific distances. These fixed orbits translate into the atom's spectrum. The atom is thus analogous to a bagpipe, which produces a series of discrete notes, even though the air movement is continuous. At least as far as the atom is concerned, the message is clear: God did not create the whole numbers. He created continuous numbers, and the rest is the work of the Schrödinger equation.
In other words, the whole numbers are not the input of the theory, contrary to what Bohr believed. They are her output. The whole numbers are an example of what physicists call an emergent quantity. From this point of view, the term "quantum mechanics" is inappropriate. Deep down, the theory is not quantum. In systems such as the hydrogen atom, the processes described by the theory form discrete growths out of a continuum standing in the infrastructure of things.
Even more surprising than this, perhaps, is the fact that the existence of atoms, and in fact of all elementary particles, is also not an input of our theories. Physicists routinely teach that the building blocks of nature are discrete particles such as the electron or the quark. This is a lie. The building blocks of our theories are not particles but fields: continuous, flowing objects spreading throughout space. The electric field and the magnetic field are two familiar examples, but there is also an electron field, a quark field, a Higgs field and many more. The objects we call elementary particles are not elementary, they are nothing but ripples in continuous fields.
The skeptics may argue that the laws of physics actually have some whole numbers. For example, the laws describe three types of neutrinos, six types of quarks (each of which appears in three forms called colors), and so on. More and more whole numbers, in every corner. indeed? In all these examples we are actually counting the number of particle types in the standard model, and it is no secret that determining this size mathematically accurately is a difficult task when the particles react with each other. Particles can change shape: a neutron can split and become a proton, an electron and a neutrino. Should we count this as one particle, three particles or four particles? The claim that there are three types of neutrinos, six types of quarks and so on is a by-product of neglecting the interactions between particles.
And here is another example of an integer in the laws of physics: the number of observed spatial dimensions is three. indeed? The late famous mathematician Benoit Mandelbrot stated that the number of spatial dimensions does not have to be a whole number. The coastline of England, for example, is about 1.3 dimensions. Moreover, in many of the unified theories in physics that have been proposed, such as string theory, the dimension of space is not unambiguous. Spatial dimensions can be created or dissipated.
I would venture to say that it is possible that only one real integer appears in all of physics. The laws of physics refer to one dimension of time. Without exactly one dimension of time, it seems that physics would become untraceable.
non-discrete ideas
Even if our current theories assume that reality is continuous, many of my fellow physicists believe that still, in this substratum of continuity resides a discrete reality. They point to examples where continuity may emerge from discrete quantities. In the macroscopic standards of everyday experience, water in a glass appears smooth and continuous. Only when you look much more closely can you see the atomic components. Could it be that a mechanism of this type lies at the root of physics? Perhaps if we look at a deeper layer, it will become clear that even in the infrastructure of the smooth quantum fields of the standard model, and perhaps even of space-time itself, there is a discrete structure.
We don't know the answer to that question, but we can glean a hint of the answer from 40 years of efforts to create a computer simulation of the Standard Model. To perform such a simulation, one must first of all take the equations expressed in terms of continuous quantities and find a discrete formulation that will fit the bits of information that the computers use. Despite efforts that have lasted for decades, no one has yet succeeded in accomplishing the task. It remains one of the most important open problems in theoretical physics, although it is not much talked about.
Physicists have developed a discrete version of quantum fields, known as lattice field theory. In this theory, space-time is replaced by a system of points. Computers estimate increments at these points to create an approximation of a continuous field. However, this technique has limitations. The difficulty lies in electrons, quarks and other material particles, called fermions. As strange as it sounds, when you rotate a fermion 360 degrees, you don't find the same object we started with. We have to rotate the fermion 720 degrees to get back the same object. Fermions resist being placed on a lattice. In the 80s, Holger Bach Nielsen of the Niels Bohr Institute in Copenhagen and Masao Ninomiya, now working at the Okaima Institute of Quantum Physics in Japan, proved a famous theorem that says that it is impossible to create a discrete model for the simplest type of fermion.
The strength of such theorems is only as strong as their premises, and in the 90s theorists, especially David Kaplan, who now works at the University of Washington, and Herbert Neuberger of Rutgers University, presented several creative methods of placing premiums on the lattice. There are many imaginable versions of quantum field theories, each with other possible types of fermions, and people are now able to formulate a model that places almost any of them on a lattice. There is only one set of quantum field theories that people don't know how to put on a lattice. Unfortunately, this group includes the standard model. We can handle all sorts of hypothetical fermions, just not the ones that actually exist.
Premiums in the standard model have a very special feature. The fermions that spin counter-clockwise "feel" the weak nuclear force, and those that spin clockwise do not "feel" it. In such a case the theory is said to be chiral (ie, lacking internal reflection symmetry). Chiral theory is a sensitive theory. Subtle effects called anomalies constantly threaten to render her internally inconsistent. Currently, such theories resist attempts to build a computer model for them.
However, chirality is not a "bug" in the standard model, which may disappear if a deeper theory is found; Rather, it is a characteristic that stands at the heart of the theory. At first glance, the standard model based on three interconnected forces seems like an arbitrary structure. Only when we think about the chiral fermions does the true beauty of the theory appear before our eyes. Like in a perfect match, with three parts that lock inside each other in the only possible way. The chiral nature of the fermions in the standard model makes all the parts fit together.
Scientists don't know for sure what the meaning of our inability to create a computer simulation of the standard model is. It is difficult to draw firm conclusions from a failure to solve a problem; It is very possible that the puzzle is extremely difficult, and is waiting for a solution that will come through accepted methods. But certain aspects of the problem echo something deeper. The pitfalls involved in this are closely related to the mathematics of topology and geometry. It is possible that the difficulty of placing chiral fermions on the lattice tells us something significant: that the laws of physics, deep down, are not discrete. We do not live in a computer simulation.
__________________________________________________________________________________________________________________________________________________________________
About the author
David Tong is Professor of Theoretical Physics at the University of Cambridge. He previously held research positions in Boston, New York, London and Mumbai. His interests focus on quantum field theory, string theory, solitons and cosmology.
Editors note
Last year, the Institute for Fundamental Questions (FQXi), in its third essay competition, presented this question to physicists and philosophers: "Is reality digital or analog?" The organizers of the contest expected the participants to decide on the digital side. After all, the word "quantum" in quantum physics means "discrete", and therefore - "digital." But many of the best articles have argued that the world is analog. One of them was David Tong's, who was one of the second place winners. The article here is an adaptation of his article.
in brief
Quantum mechanics, it is commonly thought, is a discrete theory by its very nature, but its equations are formulated in terms of continuous quantities. Discrete values emerge from it, depending on how each system is constructed.
Digital partisans insist that the continuous sizes, if we look at them closely, will turn out to be discrete: they rest on a dense grid that creates the illusion of continuity, like the pixels on a computer screen.
However, the idea of a discrete and pixelated space contradicts at least one characteristic of nature: the asymmetry between left and right versions of elementary matter particles.
And more on the subject
Quantum Field Theory in a Nutshell. Second edition. A. Zee. Princeton University Press, 2012.
Chiral Symmetry and Lattice Fermions. David B. Kaplan. http://arxiv.org/abs/0912.2560
Helicity, Chirality, Mass, and the Higgs. Flip Tanedo. www.quantumdiaries.org/2011/06/19/helicity-chirality-mass-and-the-higgs
The Foundational Questions Institute essay contest entries: www.fqxi.org/community/essay
To listen to the interview with Tong, go to the address
ScientificAmerican.com/dec2012/digital
8 תגובות
One of the interesting topics and no comments? What happened to the site?
Yaron, it seems to me that you are mixing a measured system with a wave function.
A wave function does not represent probability. It should be precise and say that its scalar product (as a result of measurement) represents a probability.
The laws of physics do not apply at all to the magnitude of the wave function. The laws of physics are smooth on the wave function itself. And the wave function itself is something continuous, it is not possible otherwise.
And what we measure does not exist by itself, but its existence depends on the wave function, that is, all the discrete existence that we see is a result that is not bound by reality of something continuous that is bound by reality.
Surely the world is continuous.
A discrete object cannot interact with another discrete object. Just like a bit cannot interact with the neighboring bit (the indirect interaction is done through a communication channel that changes voltages, but then we are already in a continuous system).
To say that something is discrete is to say that it does not interact with anything beyond itself. And the only discrete thing we know is our universe. We have universe 1 that doesn't interact with anything else.
to another one. The wave functions represent in special relativity, the spatial probability of the entity, and there they are continuous. But the eigenstates in which the particle can exist are discrete - in the deepest sense of quantum theory. The energy, the momentum, the angular momentum, and a variety of other related values.
When the system becomes multi-particle, such as fermions (for example electrons) in a crystal, then the eigenstates become continuous in the sense that they cannot be distinguished and their statistics are Fermi-Dirac and a structure of continuous energy bands is created. Within the band, all the energy values are actually obtained because it is not possible to distinguish the differences between them due to the multitude of eigenstates.
Similar to bosons (for example photons, particles that can exist more than one in the same energetic state) bands and Bose-Einstein statistics are formed. In this sense the article touched a deep truth. The difference between quantum and classical, is really at a deep level between a digital world and an analog world. Of course they will immediately come and jump that there are many more differences - true. The puzzle is open. Every time you open a layer of a doll in Bushka, the internal structure of another doll on a much smaller scale is revealed. The standard model supports 13 particles as a reminder, if gravity is included the number of particles increases. But as the energy in the LHC increases, more and more forces and more particles are revealed. They are currently in the standard model (4 forces), but already implying that there is more than a single Higgs boson.
I look forward to articles like this for a long time.
Just because something is quantum does not make it discrete in space.
Quite the opposite. Quantum theory is actually a good explanation for the fact that the universe does not consist of discrete particles in space but of wave functions.
(quantized or not). This is at the basis of quantum theory - uncertainty in space, etc...
*Somehow
It seems to me that the answer to the question of whether the world is continuous or analog is equivalent to the answer to the question of life, the universe, and everything else... and therefore I wholeheartedly say that it has nothing to do with the number 41.999999999