Weizmann Institute scientists were able to observe for the first time elastic waves in the flow of a viscoelastic fluid - in a wavelength range different from that predicted by theory
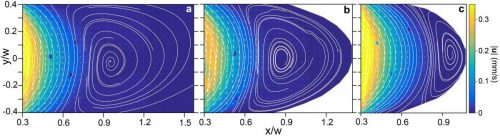
If we take large molecules of polymer, add them to the solution and make them flow, for example, by tilting the container and creating different speeds at different points, something miraculous will happen: the molecules will stretch as they move, and the solution - even if it seemed watery before - will become flexible and viscous. This phenomenon, known as viscoelasticity (or viscoelasticity if you like), results from the stress fields created by the stretched molecules. "DNA and RNA molecules or synthetic polymer molecules - they all behave exactly the same," says Prof. Victor Steinberg from the Department of Physics of Complex Systems at the Weizmann Institute of Science. About 20 years ago Prof. Steinberg and the members of his research group described a new type of chaotic flow in viscoelastic fluids, which they called Elastic Turbulence. Now they provide evidence of another viscoelastic phenomenon: elastic waves.
Elastic waves were already described years ago in the analyzes of theoretical physicists from the Weizmann Institute of Science and the Landau Institute for Theoretical Physics in Moscow. However, the theorists predicted that no one would ever see these waves, because they decay quickly in the elastic stream. "Chaotic elastic flow," explains Prof. Steinberg, "is different from, say, a flowing river, since in a river, the chaotic flow of water is random, both in space and in time, while in chaotic elastic flow, the flow is smooth in space, but random in time." Just as the elastic flow is unusual, so are the elastic waves - unlike sound waves or waves in the ocean, whose speed depends on the properties of the material, the speed of the elastic waves depends solely on the efforts exerted on the polymers while they are stretched in the current. "The closest analogy to this in our day-to-day life," says Prof. Steinberg, "is the sound of a fret on a violin or a guitar - which is influenced by the direction of the strings."
Since the discovery of elastic flow, Prof. Steinberg and his research group have performed many experiments to measure the stretch and effort of a single polymer molecule in solution. Since elastic waves were not observed in these experiments, the researchers assumed that they decayed too quickly to be recorded, as predicted by theoretical predictions.
Chaotic elastic flow is different from, say, a flowing river, since in a river, the chaotic flow of water is random, both in space and in time, while in chaotic elastic flow, the flow is smooth in space, but random in time."
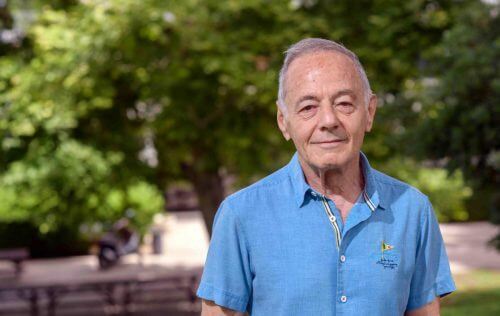
All of this changed recently when Prof. Steinberg and Dr. Atul Varshani, a postdoctoral researcher in his group, first observed elastic waves in the flow in microtubes between two obstacles with large gaps between them. "Now I understand why we did not succeed in the previous attempts," says Prof. Steinberg. "We found elastic waves in a wavelength range different from that predicted by theory - longer wavelengths with smaller decay." Since the velocity of the elastic wave is determined only by the elastic stress, this is the first direct measurement of stress in elastic flow.
The researchers also discovered that the greater the stretch in the polymers, the greater the speed of the wave - and when the stretch is relaxed, the speed drops almost completely. The waves closest to those observed by the researchers are of the type that appear in plasma when stress is applied to it through a magnetic field. The research findings were recently published in the scientific journal Nature Communications.
Apart from the theorists, who will now have to adapt the model to the new findings, the discoveries may also be relevant to other fields. Flow of polymers in tiny tubes may explain the flow properties of biological fluids in a cell. In addition, the findings may have implications for microfluidics - a rapidly growing field of knowledge, especially in the context of technological systems operating in a liquid medium.
This is how the electrons flow
Another microfluidic system in Prof. Steinberg's laboratory was recently used to demonstrate a phenomenon that, apparently, has nothing to do with liquids and flow: electric current in a two-dimensional material. The material is graphene - a single layer of carbon atoms organized in a wire-like network. The material, which was first discovered by researchers in the UK in 2004, is a semiconductor that has sparked the imagination of researchers around the world who have recognized the possibility of using it to make almost anything - from foldable electronic devices to smart paints. However, basic research in the field is still concerned with fundamental questions, such as how electrons move in this unique two-dimensional layer. Previous studies have shown that the flow of electrons in graphene is not at all similar to the flow of electrons in a copper wire - that is, unidirectional and uniform - but rather tends to behave like a viscous liquid. Other studies have shown that in certain areas of the graphene layer, the electrons can rotate and "go against the current" - similar to the eddies that appear in a viscous liquid.
Eddy flow in a rectangular space (the arrows indicate the direction of the flow)
Since it is difficult to observe electron vortices, the researchers chose to demonstrate these vortices by flowing liquids with a viscosity close to that of electrons in graphene in microfluidic tubes. Prof. Steinberg, Dr. Varshani and the former research student, Yonatan Meisel, found that in all that was said about vortices, the results of the experiment matched the theory. However, it was discovered that the similarity between electron flow in graphene and the classical model of viscous flow is even greater: the friction threshold required for the start of the electron vortices, as predicted in theory, was also verified experimentally in a viscous liquid. Thus, as reported in the scientific journal Nature Communications, it appears that the two-dimensional theoretical model of electron flow in graphene is indeed compatible with the classical model of three-dimensional fluid flow.