A new scientific field, bioinformatics, which combines biology and computer science * A robot for printing DNA chips. The blacksmith's arm collects known DNA segments and "prints" a signal on the chips
Marit Selvin, Haaretz
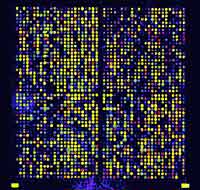
The discovery of the human genome sparked a new "gold rush" for the development of drugs that would be based on genetic information. The need to put order in the enormous amount of information and to add the pieces of information to an overall picture created a new scientific field, bioinformatics, which combines biology and computer science
A robot for printing DNA chips. The arm of the device (in yellow) collects known DNA segments and "prints" them on the chips, hundreds of millions of different combinations of the four letters - A, C, G, T. This is how the DNA map that was published about two months ago looks. In all these lies the secret of life. To the common reader, this strange map looks like a night of meaningless letters. The scientist knows that the order of the letters is not accidental, and that they represent the building blocks of approximately 26 genes that provide the operating instructions for the life processes.
But where exactly are the genes and what is each of them assigned to? Finding order within these billions of letters seems an impossible task. Even if we start from the assumption that each gene is located in a defined region of the DNA helix, it is now clear that each gene codes for more than one protein. How do you find these regions, when it is known that only about 8% of the entire genome belongs to functional genes, and the rest consists of meaningless DNA sequences whose function is still unknown?
The amount of information flowing through research laboratories is increasing. Already eight months ago, a DNA chip was in action. The different colors make it possible to identify the intensity of the activity of the genes. The company "Celera Genomics" announced that it has an amount of information that can be stored on 80 thousand CDs, which if arranged (with their covers) on shelves, the length of the shelves would reach a kilometer. But even when the location of genes on the DNA coil is discovered, the long and arduous road to cracking the genetic code will only begin. Most of the questions are still open: What is the function of each gene? When and in which tissues in the body are different genes activated? What are the proteins that these genes encode? What is the spatial structure of these proteins? How do they react to each other in health and illness?
The need to deal with the enormous amount of information, to put order in it and to understand how the pieces of information join into an overall picture, created a new scientific field called bioinformatics, a field that combines biology and computer science. Already today, about 50 private and public companies are involved in the bioinformatics business that collect the information, translate the facts and produce databases. The companies' databases include information on DNA segments that have already been decoded, where and when certain genes are activated. The information also includes tiny genetic differences between a person and their friend, the structure of different proteins and maps describing how the proteins work with each other. The databases also include the details of mapping the genetic material of other creatures that have already been discovered. Access to the information is sold to pharmaceutical and biotechnology companies for millions of dollars.
The pharmaceutical companies are interested in the databases, because with them it will be possible to find good targets for drug development. Understanding the genetic basis of a certain disease makes it possible to know what the defective protein is and to develop a medicine that will block its action.
Already today you can see the buds of the biomedical revolution following the development of bioinformatics. New drugs are produced using sophisticated techniques based on understanding the function of various genes.
This new field, called pharmacogenomics, focuses on the search for proteins that may serve as targets for new drugs. This process shortens the development time of a new product, extends the time it can be used before its patent expires, and thus greatly increases the company's profits. The rapid development in the field will lead, in a relatively short time, to the development of medicines that will be tailored according to the genetic profile of each person. Analysts estimate that the field will gain momentum towards 2005 and generate funds in the order of billions of dollars. It is therefore no wonder that giant pharmaceutical companies, academic laboratories and public funds are joining together in the virtual "gold rush" to reveal the promising information stored in the genome.
The field of drug development is undergoing a conceptual transformation these days. Until recently, the medicinal intervention was usually in the symptoms of the disease, usually through the search for new drugs from plants, or the development of substances similar to existing drugs, which mimic their mode of action. Even if the mechanism of action of a particular drug is understood, it is difficult for doctors to guess which drug will work successfully on which patient. Some drugs work well for one patient and have no effect on another. The genetic make-up of each individual is responsible for this, and understanding it will make it possible to tailor a personalized medicine to each patient.
The application of this technology is currently reserved for the future, but already today there is a dizzying development in the development of genomic drugs - drugs whose development is based on genetic information. Pharmaceutical companies are constantly looking for genes that code for proteins that may participate in disease processes. If a gene is found that has been proven to participate in pathological processes, the bioinformatic databases are searched for similarities between the gene's DNA segment and DNA segments from other organisms, whose sequence is known and the protein encoded by it is also known. The logic of such a search is based on the fact that parts of the DNA, which code for proteins that participate in important life processes, are usually preserved during evolution and their sequence is similar in yeast, flies, rodents and humans. Therefore, it is possible to compare the different sequences, and from the similarity between the genes, it is possible to conclude about the nature of the protein that the gene encodes, and sometimes also about its structure and mode of action.
One of the examples of this is the innovative development of a drug against osteoporosis - a disease that manifests itself in the depletion of bone tissue and attacks many women at the age of menopause. In 1993, researchers from the American pharmaceutical company "Smith-Kline-Bizim" found a gene that was isolated from a certain type of bone cells. These bone cells are broken down in the normal process of building and breaking down the bone, but in osteoporosis patients the breaking down activity is increased, which causes the bone tissue of the patients to deplete. The researchers turned to scientists from the bioinformatics company Human Genom "Sciences" and asked them to determine the DNA sequence of the gene and search their database for sequences similar to it, in order to give them some clue about the nature of the protein that the gene encodes.
The DNA sequence of the gene was identified in the databases and a sequence similar to it was also found; This made it possible to identify the protein that the gene encodes. It turned out that the protein belongs to a group of known molecules, cathepsins.
This exercise in bioinformatics gave scientists
"Smith-Klein-eggs" within a few weeks a promising goal for the development of a new drug that will inhibit the activity of the protein and possibly slow down the rate of bone breakdown. Needless to say that in the standard laboratory experiments, the possibility of reaching such an achievement requires many years of hard work, and not always with success. Now the company's scientists are trying to develop a drug that will bind to the active site in the cathepsin molecule and inhibit its activity. These experiments are still conducted in a "wet" laboratory, that is, in classical experiments in which the activity of the drug, its toxicity and the extent of its absorption in the body are tested - in laboratory experiments, in animals and in human clinical trials.
Here, too, bioinformatics helps researchers. Scientists believe that the day will not be far away when, based on data such as protein structure and interactions between different proteins found in the databases, it will be possible to computerize all the indicators tested today in the laboratory. This revolutionary work method is called "biology in silicon".
Alfred Gilman from the University of Texas Medical Center, winner of the Nobel Prize in 1994, is already trying to implement this. With the help of 50 researchers from different universities, he is developing a "virtual cell" in a computer, in which information about the chemical communication systems in the living cell will be encoded. This electronic cell will make it possible to test the new drugs in development in the future without the need for experiments on animals and humans.
These steps are the beginning of the revolution in the development of genomic medicines. An entire industry is now developing to take advantage of the information stored in genes, to check when and in which tissues the genes become active and to identify the properties of the proteins that these genes encode. The identification of the proteins encoded by the genes, and the understanding of their mode of action, created a new field that engulfs many companies and investments of hundreds of millions of dollars, called "proteomics". What is interesting today in the genome, researchers admit, are not the genes, but what can be done with them.
One of the technologies that examines this is the "DNA chips" technology. In one of its most important applications, this technology locates the active genes in the cell. Using it, it is possible to trace, for example, the unique characteristics of cancer cells compared to healthy cells. Cancer cells activate genes that contribute to the cancer process and its various characteristics. Different types of cancer, and even tumors of the same type, have a unique genetic profile that can explain their degree of violence and response to different treatments. Locating these genes will provide important information about the nature of the cancer process and will make it possible to develop substances that will thwart it.
The DNA chips are small chips the size of a coin, divided into tiny boxes in which thousands of DNA segments from known genes are placed side by side. These genes are compared with genes from certain tissues. Using a special method, molecules are extracted from the tissue that represent the genes active in it and turn them into DNA molecules known as "complementary DNA". This DNA is marked with a fluorescent substance. When the complementary DNA molecules are brought into contact with the chip, each of the genes in the tissue being tested adheres to its corresponding gene on the chip and a lighted dot is obtained. According to its location and the intensity of the light, it is possible to identify, through a computerized scan, the identity of the gene and the extent of its activity. In the past, DNA chips were expensive, but today new technologies make it possible to produce them at a low price, which makes them available to the diagnostic market.
Using the DNA chips, they tried to understand why in the blood cancer of the lymphoma type, 40% of patients respond to treatment and 60% succumb to the disease. Testing DNA samples of lymphoma patients of a certain type using DNA chip technology showed a large difference in the active genes between the two groups. The analysis of the results on the computer revealed that it is possible to divide the patients into two groups according to the degree of activity of certain genes.
One group responded to chemotherapy treatments, compared to the other group that did not, and the degree of survival of the patients also differed from group to group. These findings have practical significance. Lymphoma patients today receive chemotherapy treatments, and when these are unsuccessful, they are transferred to a bone marrow transplant. In the future, lymphoma patients with a genetic profile indicating a lack of response to chemotherapy treatments will be immediately referred for a bone marrow transplant, saving unnecessary suffering and damage.
Using the DNA chips, different activity patterns of genes were also found in melanoma (violent skin cancer). Using DNA chips, researchers compared the active genes in violent melanoma tumors that metastasize with high frequency, with less violent tumors. The comparison revealed several genes whose activity increased when the melanoma cells began to metastasize. Most of these genes are related to the cells' ability to move and penetrate new tissues. Even in breast tumors, which apparently present the same pathological picture in patients, there are differences in the activity pattern of many genes, which is probably responsible for the different response to treatments between patients.
These studies are the first examples of the beginning of branch research to clarify the chain of genetic events leading to the development of many cancerous conditions and to develop an approach to treatment adapted to the patient, an approach that will provide an accurate genetic analysis of the patients and offer a treatment "tailored" to the unique genetic profile of the disease.
At the National Cancer Institute in the US, they have been researching the genes and the proteins they encode that are present in cells of different types of cancer for the past two years. The project, called the "genetic index of human cancer", is a collaboration between the American government, universities and large pharmaceutical companies. So far, more than 50 genes active in different types of cancer have been isolated. For example: 5,692 genes were found that are active in breast cancer cells, of which 277 genes are inactive in other tissues. Proteins directed against the products of these genes could be used as unique drugs for breast cancer alone without causing side effects.
"The post-genomic future is already here and now, and it changes many aspects of our view of the individual," concludes Prof. Hermona Sorek from the Institute of Life Sciences at the Hebrew University.