A thought experiment from the 19th century became a real technique for achieving very low temperatures, paving the way for new scientific discoveries and useful applications
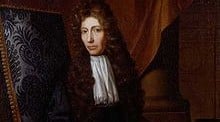
As you read this article, the air molecules are flying around you at 3,000 kilometers per hour, faster than a bullet, bombarding you from all sides. At the same time, the atoms and molecules that make up your body are constantly swirling, vibrating or colliding with each other. Nothing in nature stands still, and the faster things move, the more energy they carry; We are able to feel this total energy of the atoms and molecules, and call it "heat".
Although perfect immobility, a state corresponding to absolute zero temperature, is physically impossible, scientists have managed to get closer and closer to this final limit. Strange quantum phenomena begin to emerge in these extreme realms and create new and unusual states of matter. In particular, cooling gas clouds of atoms, as opposed to matter in a solid or liquid state, and bringing them to the high temperature of only a tiny fraction of a degree above absolute zero, has given researchers the ability to observe particles of matter behaving like waves, create the most accurate measuring devices in history, and build the most accurate opaque clocks .
These methods of cooling atoms have a drawback: they can only be applied to a few elements from the periodic table and therefore their effectiveness is limited. For example, cooling hydrogen, the simplest atom, has long been an extremely difficult challenge. But not long ago my research group demonstrated a new cooling method that works on most elements and also on many types of molecules.
My inspiration was a thought experiment by Victorian-era James Clerk Maxwell. This great Scottish physicist brought up in his mind the conceptual existence of his "demon" that actually existed, it seems that he has the power to break the laws of thermodynamics.
The recently discovered cooling capacity will open new directions for fundamental research and lead to a wide range of practical uses. For example, some versions of the technique may lead to processes for refining rare isotopes that play important roles in medicine and basic research. It could also have other side effects, such as improving the accuracy of nanoscale methods used to make computer chips. And scientifically, cooling atoms and molecules can allow researchers to explore the twilight zone between quantum physics and ordinary chemistry, revealing possible differences between the behavior of matter and antimatter. Also, supercooling of hydrogen and hydrogen isotopes could help small laboratories to answer questions in fundamental physics, of the type that until now required huge experiments such as the experiments conducted in particle accelerators.
Bullets racing
Stopping atoms and molecules and controlling them is not a simple task. In a typical experiment, the researchers' first step is to produce a thin gas from some chemical element, by heating a solid or vaporizing it with a laser. In the next step, the gas must be slowed down, sealed in a vacuum chamber and kept away from the chamber walls.
My first step was an old trick. More than 40 years ago, chemists discovered that a gas at a pressure of several atmospheres escaping through a small hole into a vacuum cools considerably as it expands. The uniqueness of these "ultrasonic beams" is that their energy is almost uniform, that is, the speed of the molecules will be very close to the average. For example, if a beam goes out at a speed of 3,000 km/h, the molecules in it will deviate from this speed by at most 30 km/h. In contrast, air molecules at room temperature, whose average speed is 3,000 km/h, can have any speed between zero and 6,000 km/h. This means, from a thermodynamic point of view, that although the beam has a considerable amount of energy, it is nevertheless incredibly cold. Think of it this way: an observer traveling alongside the beam at a speed of 3,000 km/h would see the molecules moving so slowly that the temperature of the beam would be no more than a hundredth of a degree above absolute zero!
I realized that if my colleagues and I could slow down and stop such a beam while preserving its narrow range of speeds, we could end up with a fairly cold collection of atoms, and then we could trap them and cool them even more.
To achieve this goal, my group began working with ultrasonic beams in 2004, in collaboration with Uzi Even, a chemist from Tel Aviv University. Initially we tried to build a propeller whose speed of movement of the tips of the blades is half of the speed of the supersonic gas beam. Direct pulses from the beam towards the retracting blades of the propeller so that the speed of the beam is exactly offset with the speed of the blades. As the gas atoms were ejected from the propeller, the propeller extracted all the kinetic energy from them, just as a tennis racquet retracted can bring a ball to rest.
But this setup was difficult to work with, as it required excruciatingly precise tuning. Robert Hebner, director of the Center for Electromechanics at the University of Texas at Austin, proposed another scheme: to cause gas to spray from the back of a projectile as the projectile sped down a coiled rifle. A coil gun (also known as a "Gauss gun") is an experimental weapon that propels magnetized projectiles out of a gun barrel using magnetic fields instead of gunpowder. It works by accelerating the projectile through a series of coils of wire through which an electric current flows, so that magnetic fields are created within them. The bullet, which is essentially a magnetic strip, is attracted to the center of the coil through which it passes. An approaching projectile is therefore accelerated by gravitational forces. However, as soon as the projectile passes the center, the forces will begin to pull it back and thus slow it down and return it to its original speed. But if you stop the current in each coil at the exact moment when the bullet passes its center, the magnetic forces always push the bullet in the right direction - down the barrel.
I quickly realized that we could implement the idea of the banner and at the same time completely get rid of the bullet. Instead we will use the same principle on the beam itself, but in reverse: this time the coils of the gun will not accelerate the projectile but will act directly on the gas molecules and bring them to rest [see box on the next page.] This trick is possible because most atoms have at least a small amount of magnetism, and they all have magnetism when their electrons are in an excited state. Many types of molecules are also magnetic.
We built the new device and tested it first on excited neon atoms and then on oxygen atoms. We managed to stop both types. Unbeknownst to us, a group working in Zurich, Switzerland under the direction of Frederic Merck had separately developed the same idea and succeeded in arresting hydrogen atoms at about the same time that we were performing our experiments. Several groups around the world have already built their own atomic coil guns, which are actually very simple and stable devices, based on ordinary copper wire, off-the-shelf capacitors and transistors.
Once we were able to stop atoms in this way, the way was pretty much paved for trapping them in static magnetic fields. The more difficult problem was finding a way to cool them even further. Although a temperature of 0.01 degrees Kelvin (one hundredth of a degree above absolute zero) sounds pretty cool, it is a long way from the limits achieved by other techniques. We therefore had to find a way to achieve an even lower temperature.
one way roads
I pondered general cooling methods long before anyone came up with atom coil guns, but for a long time I didn't find a solution. The technique of laser cooling, invented in the 80s, was very successful and resulted in the creation of a state of matter known as the Bose-Einstein condensate and the awarding of two Nobel Prizes in Physics in 1997 and 2001. But the range of applications of laser cooling is mostly limited to atoms in the first column of the periodic table, such as sodium and potassium, because it is easy to excite the elements from the ground state to a single excited state, as the technique requires. Another method I considered was evaporative cooling, which relies on collecting the hot atoms and leaving the colder atoms behind (this is the principle by which sweat cools our bodies as it evaporates from our skin). But without the help of laser cooling, it is very difficult to achieve a high enough density to start the evaporation in the first place.
In February 2004 I visited Princeton University and spoke there with Nathaniel J. Fish, a physicist who deals with plasma. He told me about an idea he developed just then: how to transmit an electric current of electrons in plasma - a gas of electrons and positive ions - using a trick that makes the electrons move in one direction and not the other. I asked myself if we could achieve a similar result using atoms or molecules: build a "gate" that allows atoms to pass in one direction but not in another.
Let's put aside the technical issue for a moment, how to actually build a one-way gate, and try first of all to understand why such a device can help with gas cooling. The first step would be to reduce the volume of the gas without raising its temperature. Suppose we have a tank separated into two volumes by a gate. Gas atoms in a tank splash in random directions randomly and sooner or later will fly towards the gate. If the gate only allows them to pass in one direction, for example from left to right, then eventually all the atoms will be concentrated on the right side of the container. The crucial thing is that the velocities of the atoms do not change during the process, so the gas will remain at the same temperature as at the beginning. (From a thermodynamic point of view, this procedure is completely different from compressing the gas to the right half of the volume, an action that causes the atoms to accelerate and therefore raise the temperature.)
The next step would be to allow the gas to expand back to its original volume. As a gas expands, its temperature drops, which is why spray cans cool down when in use. The end result will therefore be gas in the original volume but at a lower temperature.
The problem that has puzzled physicists for a long time is that such atomic sorting gates seem to violate the laws of physics. When the gas is in the compressed state, its entropy is low (entropy is a measure of the amount of disorder of a system). But according to the second law of thermodynamics it is impossible to lower the entropy of a system without wasting energy and creating more entropy elsewhere.
Many pens have been written about this paradox since the thought experiment presented by James Clerk Maxwell in 1871, in which "a wise and nimble being" can see the movements of particles, and open or close a gate accordingly. This hypothetical creature became known as "Maxwell's Demon" and appears to violate the second law of thermodynamics, as it is able to lower the entropy of a gas while expending a negligible amount of energy. After many years, in 1929, Leo Szilard solved the paradox. He raised the possibility that the demon was gathering information every time the gate was closed. This information, according to him, carries an entropy that precisely balances the decrease in entropy of the gas, and thus "saves" the second law. (Szilard was ahead of his time: a few decades later, it was the idea that information had actual physical meaning that motivated the creation of information theory.)
All the discussions on the subject of Maxwell's dilemma, including Szilard's solution, did not go beyond the theoretical hypothesis, and for many decades it seemed that they were destined to remain so. But my colleagues and I created the first realization of Maxwell's thought experiment in the way Maxwell imagined it. (In other recent experiments similar things were done at the principle level, but using nanomechanics and not using gas gates.) And we used this method to cool atoms to a temperature of 15 millionths of a degree above absolute zero.
As we will see later, the device we built clarifies how Maxwell's demon can actually exist, as well as why Szilard's insight - that information plays a crucial role - is correct.
I concluded that if I want the one-way gate to work, the gas atoms need to have two distinct states (different values of electrons around the nucleus) that are both low energy and therefore stable. Let's call the two states "blue" and "red". The atoms float in a container that a laser beam crosses in the middle. The wavelength of the beam is precisely adjusted to a size that will cause "red" atoms to bounce back as they approach the beam, that is, the beam essentially functions as a closed gate. At first all the atoms are "blue" and therefore can pass through the laser barrier without interference. But just to the right of the blocking beam, the atoms are hit by a second laser beam, adjusted so that atoms turn from "blue" to "red" by scattering a single photon. Now the "red" atoms are repelled by the blocking beam and therefore cannot pass through the gate and return to the left side. Eventually all the atoms will collect on the right side, and the left side will remain empty.
We first demonstrated our gate using rubidium atoms in early 2008. We called our method single-photon cooling, to distinguish it from the earlier method, laser cooling, which requires many photons to cool each atom.
Meanwhile, unbeknownst to us, Gonzalo Moga from the University of Bilbao in Spain, together with his colleague Andreas Ruchhaupt (now at the Leibniz University in Hanover, Germany), developed a similar idea without knowing our research. Since then, Moga, Rochaupt and I have mathematically analyzed some of the theoretical aspects of the gate. In a joint paper published in 2006, we showed that when an atom emits one photon, the photon carries with it information about that atom and therefore also a tiny amount of entropy. Moreover, the original photon is part of an ordered beam of photons (the laser beam), while the scattered photons are scattered in random directions. The photons thus become less ordered, and the corresponding increase in the entropy of the light precisely balances the decrease in the entropy of the atoms, which results from the barrier created by the one-way gate. Thus, single-photon cooling acts like Maxwell's demon in exactly the way that Leo Szilard envisioned in 1929. The demon, in this case, is simple and extremely effective: a laser beam that induces an irreversible process by scattering a single photon. Such a demon is certainly not an intelligent being nor a computer, and does not need to make decisions based on information coming from the atoms. The fact that the information is available and can, in principle, be collected is sufficient.
The capture and cooling front
The control of the movement of atoms and molecules opens up new directions in science. Chemists have long dreamed of trapping and cooling molecules to study chemical reactions governed by the laws of quantum mechanics. The coil gun works on any magnetic molecule and provides a complement to the method that uses electrical, rather than magnetic, forces to slow down any electrically polarized molecule. If the molecules are small enough, the single-photon cooling can lower the temperatures enough for quantum phenomena to start to dominate. For example, molecules become propagating waves that can cause reactions over much greater distances than usual, without needing the kinetic energy that fuels normal reactions. Several research groups are now delving into this direction.
Single photon cooling has another huge advantage: it works on hydrogen and also on its isotopes deuterium (whose nucleus has a neutron in addition to the single proton) and tritium (with two neutrons). In the late 90s, Dan Klefner and Thomas J. Greatek of the Massachusetts Institute of Technology (MIT), after great efforts, succeeded in capturing hydrogen and cooling it using cryogenic methods and evaporative cooling, but they never achieved a similar feat with other isotopes. Further progress depends on new methods to capture and cool hydrogen isotopes in a relatively simple device. Single-photon cooling is remarkably well suited for trapping and cooling all three isotopes of hydrogen. One goal will be to stretch the current limits of super-precision spectroscopy, another important application of cold atoms.
Capturing and cooling tritium may make it possible to measure the mass of the neutrino, the most common elementary particle in the universe among the known particles, and thus better understand the gravitational effects of these particles on the evolution of the cosmos. Tritium is radioactive, and turns into helium 3 when one of its neutrons decays and becomes a proton, an electron, and an anti-neutrino, the antimatter particle corresponding to the neutrino. By measuring the energy of the electron, which is fired from the nucleus in the form of beta radiation, the physicists can determine how much energy the anti-neutrino took with it, which passed through the device without being detected, and from that deduce what its mass is. Physicists expect the mass of the neutrino to be the same as the mass of the antineutrino.
The same methods can be used to trap and cool antihydrogen, the antimatter equivalent of hydrogen. Antihydrogen was only recently created at CERN, the physics laboratory near Geneva, and must be handled with extreme care since antimatter disappears in a flash of energy as soon as it comes into contact with matter. In this case, the ultrasonic beam method cannot be used as a starting point. Instead, it is possible to create a beam of anti-hydrogen particles by launching anti-protons through a cloud of positrons and then stopping and cooling it with the Maxwell demon we created. Experimenters studying antihydrogen will be able to answer the simple question: "Does antimatter fall in the same way as matter?" In other words, "Does gravity act in the same way on all bodies of the same mass?"
The new atomic coilgun and single-photon cooling techniques may also have important practical applications. Isotopes of most elements in the periodic table are still separated using a device known as a calutron, invented by Ernest Lawrence during the Manhattan Project. Clotrons separate the isotopes, whose masses differ slightly from each other, with the help of an electric field, more or less like a large mass spectrometer. Today there is only one active Klotron program, in Russia, and it is not really effective. In this case it is possible to design a Maxwell demon similar to a demon that operates under cooling that can separate isotopes in the beam more efficiently than protons. With this method it is possible to produce small amounts of isotopes, such as calcium 48 or ytterbium 168, elements that play a role in medicine and basic research, without increasing the risk of nuclear proliferation because this method is only practical for isolating very small amounts of isotopes.
Another side effect we are testing is the construction of structures on a nanometer scale. Instead of using magnetic fields to slow down atoms, we could allow the fields to focus beams of atoms like lenses focus light, but with a resolution of only one nanometer or even less. In this way, such beams will be able to sink atoms and create smaller details than is possible today with optical lithography, the gold standard of computer chip manufacturing. The ability to create structures on a nanometer scale with such a method, from the bottom up, rather than the top-down approaches, which are more common in the nanosciences, will establish a new field of research that I call atom-science.
Even if absolute zero remains as unattainable as ever, there is still much to discover, and gain, in our journey on the road leading to it.
______________________________________________________________________________________________________________________________________________________________________________________
About the author
Mark G. Raizen holds the Sid W. Richardson Foundation Administrator's Chair in Physics at the University of Texas at Austin, where he also received his Ph.D. His interests include optical trapping and quantum entanglement. When he was a toddler, Raisen got to meet Leo Szilard, who was a patient of his cardiologist father, and explained why Maxwell's demons do not violate the laws of thermodynamics.
in brief
Traditional methods for cooling gases down to a temperature close to absolute zero work only on a few fundamentals.
A combination of two innovative techniques can cool atoms of almost all elements and even some molecules.
One of the techniques, which seems to violate the second law of thermodynamics, is a physical realization of a famous thought experiment from the 19th century, known as Maxwell's Demon.
Cooling: the first step
Magnetic brakes
The first step of cooling can lower the temperature of a gas to about a hundred degrees above absolute zero by shooting the gas into a vacuum at high speed (a process that causes the temperature to drop significantly) and then slowing it down with a new device known as an atomic coil gun. Coilguns were originally used as experimental weapons built to accelerate projectiles using magnetic fields. The atomic spiral gun applies the same idea in reverse to slow down any atom or molecule with a north and south magnetic polarization, a property that most elements in the periodic table have.
The applications of the method extend from the study of the properties of elementary particles without the need for expensive accelerators to the separation of isotopes for use in medicine and research.
Cooling: the second step
Demonic cold
After an atomic coil gun or other device has cooled gas to a temperature of a few hundredths of a degree above absolute zero, one can begin the serious freezing, down to millionths of a degree and even less than that. The new technique of single-photon cooling succeeds in this task by a one-way gate inspired by a 19th-century thought experiment. The idea is first of all to allow the gate to concentrate atoms in a small volume (but without raising their temperature) and then to allow them to spread back to their original volume (a process that lowers their temperature).
And more on the subject
The Spectroscopy of Supercooled Gases. Donald H. Levy in Scientific American, Vol. 250, no. 2, pages 96–109; February 1984.
Demons, Engines and the Second Law. Charles H. Bennett in Scientific American, Vol. 257, no. 5, pages 108–116; November 1987.
Laser Trapping of Neutral Particles. Steven Chu in Scientific American, Vol. 266, no. 2, pages 70–76; February 1992.
11 תגובות
Great article!
Can the one-way atomic gate method be used on a larger scale?
In such a case it is possible to produce a heat pump (air conditioner) with minimal energy consumption.
If gas can be compressed in a tank using this method, slowly but surely, the barrier of the Carnot cycle can be broken.
Is this theoretically possible or is there some limitation? And how is the information expressed about the position of the electron energetically? To me it sounds like a minimal loss of energy...
Following Ehud's words - here is the news from "Haaretz":
http://www.haaretz.co.il/hasite/spages/1230499.html
The mention of anti-hydrogen capture in the article has an Israeli connection. One of the researchers on the team that managed to capture
Anti-hydrogen is an Israeli scientist, Dr. Eli Sharid. Just today it was reported by Pratlamon ("Haaretz") that the researchers of Team Alpha
who are responsible for trapping the anti-hydrogen in the axis have managed to extend the duration of the trapping of this anti-atom to a chapter
Time of 15 minutes. This period of time is 6000 times longer than their previous achievement from a few months ago which was chosen as the most important scientific breakthrough in 2010 by Physics World.
Anat:
You should read the article carefully before you try to present the this person As one who does not understand what he is talking about.
I was convinced by your words of only one thing, and that is that you do not know what he is talking about.
Mark J. Risen apparently didn't learn lesson number 1 in physics as the wavelength gets colder and longer.
Therefore, there is no connection between nanometer lithography and supercooling.
It's like crossing a banana with a mammoth. Or more like trying to thread a monkey through the eye of a needle it's possible but that's not it.
to her daughter,
When you receive the soul-creative, don't forget to send me some with your brother Guy Ben Hanom, okay?
Just make sure it doesn't melt.
A happy article, especially in light of the fact that after they succeed in freezing the gases, and the other particles, there will also be someone who will succeed in freezing souls, and we will finally be able to know the truth about the essence of the soul and its role.
Yes, very interesting, thanks too.
Very interesting article.
Thanks 🙂
Article from A-V-L-E
In other words, we have two stages here: in the first stage - the cold of dogs, in the second stage - the cold of demons.