Evolutionary biologists are trying to fight bacteria in a new way: destroying their social life
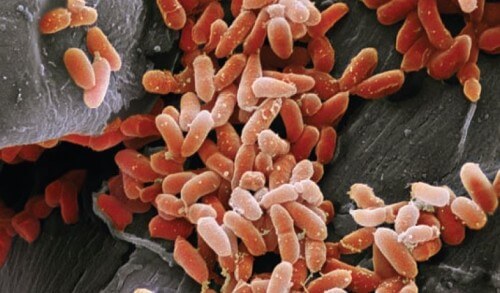
Rolf Komerli researches new drugs to treat deadly infections. He spends his days in a laboratory full of petri dishes and bottles of bacteria - exactly where you would expect to find him. But Komerli came to this laboratory at the University of Zurich in a strange way. As a PhD student he spent years traveling in the Swiss Alps to study the social life of ants. Only after he received his doctorate in evolutionary biology did he turn his attention to bacteria.
The route from ants to antibiotics is not as winding as one might think. For decades, scientists have studied how cooperative behavior developed in animal societies such as ant colonies, where sterile females work to raise their queen's eggs. A new branch of science, sometimes called "sociomicrobiology", suggests that some of the principles that govern ants' behavior can explain the emergence of bacterial societies. Like ants, bacteria also live in complex communities, where they communicate with each other to cooperate for the common good. This insight into the social evolution of bacteria suggests a new strategy to fight infections: instead of attacking individual bacteria, as traditional antibiotics do, scientists are testing the possibility of attacking entire bacterial populations.
And new strategies are now needed. Bacteria have developed widespread resistance to antibiotics, and medicine is in crisis. For example, the US Centers for Disease Control and Prevention (CDC) estimates that 23,000 people die in the US each year from antibiotic-resistant infections. There are strains of tuberculosis and other pathogens that are resistant to almost every drug. "It's a big problem," says Anthony S. Fauci, director of the American Institute of Allergy and Infectious Diseases. "And there is every reason to believe that the situation will only get worse."
The common response to the crisis was to slow down the evolution of resistance and find new drugs to replace the weakened ones. Bacteria develop resistance relentlessly and will continue to do so unless we find a different way to fight them. "Every time we develop a new drug it fails," says John Pepper, a theoretical biologist at the American Cancer Institute. "And so the solution is, 'Hurry! We will open another antibiotic!' It is effective for a few months, but it is no longer good enough."
The ability of many of the infectious strains of bacteria to make us sick depends on the general behavior. Sociomicrobiologists will look for opportunities to disrupt bacterial society, for example by impairing communication or blocking the bacteria's collective efforts to gather nutrients. According to evolutionary theory, the collective behavior of bacteria should be an excellent target for drugs. An attack on social life is obviously not completely immune to evolution. But at the very least it may slow down the development of resistance to a considerable extent.
Sociomicrobiologists have to overcome a lot of skepticism. Although they have presented detailed theoretical arguments and some promising experimental results, some researchers doubt the ability of evolutionary-inspired drugs to stop the development of resistance. And the pharmaceutical companies, which are moving away from the development of antibiotics in general, are still not ready to promote such drugs throughout the approval and marketing process.
And yet, sociomicrobiologists are gaining attention. The US Institutes of Health (NIH) have formulated programs for the study of antibiotic resistance, and researchers are making the bacterial community a top priority. If the hopes come true, these studies will succeed in reversing the relationship between medicine and evolution. Evolution, traditionally an enemy in the war against germs, will become a friend.
The evolution of drug resistance
The antibiotic resistance crisis has been developing for a long time. A few years after the first antibiotics entered the market, in the middle of the 20th century, doctors already discovered some bacteria that were resistant to them. At the time it was not entirely clear what was happening. Today, of course, scientists can study the evolution of resistance in all its molecular components.
Penicillin, for example, kills bacteria by binding to a protein that helps bacteria build their cell walls. Without this protein the bacteria leak and die. In every population of bacteria there are some that are able to protect themselves from penicillin thanks to a mutation they have passed on. For example, bacteria have pumps that pump toxic chemicals out of the bacterium. A mutation may give the bacterium the ability to produce more pumps and thus get rid of the penicillin quickly and allow its proteins to produce the cell wall.
Under normal circumstances such mutations do not provide an evolutionary advantage to bacteria. But if a patient is taking a dose of penicillin to fight an infection, suddenly the extra pumps can provide a huge advantage. Bacteria without the extra pumps die, while those that have undergone this mutation continue to survive. The survivors reproduce and increase their proportion in the population. In future generations, their offspring, who carry the original mutation, may develop even better defenses, sometimes through the transfer of genes from other bacterial strains.
In the course of decades, new drugs were developed at a fast enough pace to replace the old drugs that failed. But now the drug pipeline is emptying. Development expenses for new antibiotic drugs are eating into profits, and many drug companies have fled the antibiotic business and started investing in more profitable drugs for cancer or jaundice.
As the crisis deepened, scientists yearned for antibiotics that would not become obsolete. And sometimes they did discover what seemed to be an evolution-proof cure. In 1987, for example, Michael Zasloff, then at the NIH, discovered that the skin of medical frogs has a powerful poison against bacteria. Zasloff and other researchers soon discovered that amphibians were not the only producers of the poison. Almost every animal they tested produced small proteins with a positive electrical charge that could kill bacteria. This group of proteins was eventually named antimicrobial peptides.
In review articles and in the news, Zasloff claimed that it was unlikely that bacteria would develop resistance to the drugs. Animals, he said, have been using the antimicrobial peptides for hundreds of millions of years, and today's bacteria are still sensitive to them. In 2003, Graham Bell, an evolutionary biologist at McGill University, predicted that Zasloff would be deceived. Penicillin and many other drugs were also discovered in nature, but modern medicine provides them to patients in huge concentrations, therefore it creates a strong evolutionary pressure that causes the increase of resistance mutations. Once doctors start giving patients pills loaded with antimicrobial peptides, history will repeat itself.
Zasloff set Bell a challenge, to test whether bacteria would develop resistance to pexiganan, one of his most studied peptides. Bell and Gabriel Perron, who was his doctoral student at the time, grew E. coli bacteria and exposed them to a low dose of pexiganan. Then they took some of the bacteria that survived, started a new colony with them and exposed it to a higher dose of the drug. They increased the dose over several weeks and watched as bacteria developed that were completely resistant to pexiganan, just as Bell had predicted.
Zasloff immediately admitted that Bel was right. The experiment made him much more cautious about the antimicrobial peptides. "If something like this can happen in a test tube, it is very likely that it will also happen in the real world," Zasloff told the journal Nature.
Today we do not know for sure if this is indeed true and we will not know unless antimicrobial peptides are finally approved for use. Currently the drug companies are conducting several clinical trials, but 28 years after their discovery, not a single antimicrobial peptide has been approved for the treatment of infections. They are victims of a slow drug pipeline.
Cooperation between bacteria
Charles Darwin could not have known that bacteria would be one of the most successful examples of his theory of natural selection. He and other scientists of his time knew very little about how bacteria grow. In 1859, when he presented his theory in his book "On the Origin of Species", he did not write about bacteria but about features that were known in the Victorian period, such as mammalian fur and feather colors.
Darwin also wrote about common natural features that first made him fear that his idea was wrong. One of them was that many active ant species are sterile. According to Darwin's theory, natural selection arises from the competition between individuals for survival and reproduction. But worker ants, who do not breed themselves, seem to have completely dropped out of the competition. Their very existence, wrote Darwin, seems "fatal to my whole theory."
Darwin suspected that the solution to the worker ant paradox lay in family ties. An ant colony is not a random collection of strangers. She is more like an extended family. Genetically related ants can create more offspring together than would have resulted from each ant's attempts to reproduce on its own.
Darwin's ideas about cooperation inspired generations of evolutionary biologists who continued to study them. This is how Comerly began his scientific career. For example, he sequenced the DNA of ants in different nests to see how genetic proximity affects their behavior towards each other. The research was fascinating but slow and limited in scope. As Comerly neared the completion of his Ph.D., he became acquainted with several evolutionary biologists who had switched from the study of social animals to social bacteria.
The words "social" and "germ" may not be associated with each other in most people's minds, but it turns out that bacteria live in intimate communities that are teeming with communication and cooperation. Take for example the bacterium Pseudomonas aeruginosa, a strain that can cause severe pneumonia. When one such bacterium invades a host, it launches signaling molecules. Members of our species can receive these signals with the help of special receptors. Sending these molecules and absorbing them is the bacteria's way of saying, "I'm here. Is there anyone else here like me?"
If the bacteria feel that there are enough friends around them, they begin to cooperate in building a shelter. They spray out sticky molecules that produce a kind of mat in which the bacteria are embedded. This surface, known as a biofilm, can stick to the walls of the lungs or other organs. Deep within the surface the bacteria are protected from attack by the cells of the immune system.
Pseudomonas bacteria also cooperate in collecting food materials. Bacteria cannot grow without iron, for example, but it is difficult to find free iron in the human body, because the cells grab it and trap it inside hemoglobin and other molecules. To get a supply of iron each bacterium releases molecules called siderophores that are able to steal iron atoms from our molecules. "In effect [the bacteria] steal iron," says Sam Brown, an evolutionary biologist at the University of Edinburgh. They can now absorb the iron-bearing siderophores and use the iron to grow.
This is a deep cooperation, because every siderophore absorbed by any bacteria was probably produced by one of its millions of neighbors. "One cell pays a price for the benefit of the entire colony, not just for its own benefit," says Pepper from the American Cancer Institute. Evolutionary theorists call such molecules public goods because they benefit the general public, in this case the bacterial community. They are the opposite of a private good, which provides benefit only to the microbe that produces it. A public good represents a Darwinian paradox, because in principle natural selection should suppress it. Bacteria whose mutation has disrupted their ability to produce a public good themselves can use the one produced by others. This inequality should result in an evolutionary advantage for exploiting bacteria. A bacterium that does not produce siderophores can get iron without paying the cost of producing them. It should multiply faster than cooperative bacteria and become more common. And yet the cooperative bacteria are common in strains like P. aeruginosa, not the exploiters.
In the first decade of the 2000s, a small group of evolutionary biologists began to consider these intriguing questions in the social life of bacteria. The University of Edinburgh stood out as a leading center for sociomicrobiology, so Comerly applied there in 2007. But he did not immediately engage in experiments. Years of studying ants did not prepare him for the hard work involved in microbiology. Comarelli and other trainee sociomicrobiologists had to be trained in the methods of microbiology. They learned how to grow bacteria, how to prevent the cultures from becoming contaminated, how to change the bacterial genes and how to conduct experiments. "I needed several years to learn all the methods," says Comerly. "And sometimes the classic microbiologists didn't take us seriously."
Eventually they got results. They began to uncover tricks by which the cooperative bacteria prevent the exploiters from reproducing. For example, in a joint study with Brown, Comarelli discovered that Pseudomonas bacteria do not produce a continuous stream of siderophores, but release them in a sudden burst. Once the bacteria release the siderophores, they can recycle the molecules. They absorb iron-laden siderophores, remove the iron atoms, and then spit the siderophores out again. Thanks to the durability of the siderophores, the bacteria do not need to invest much energy in producing new siderophores to replace the old ones. The cycle reduces the cost of cooperatives. It also reduces the utility of exploitation.
As sociomicrobiologists studied the social evolution of bacteria, they wondered if they could apply their insights in a practical way: finding new drugs to fight infections.
turning point
For the evolutionary biologist, all the antibiotics we use are the same. They all attack the private goods of the bacteria. If a bacterium mutates to protect its private goods, it will win the competition against the normal bacteria. Sociomicrobiology points to a different goal for stopping infections. "Instead of attacking individual cells, one should attack their public goods," says Pepper. Evolutionary theory predicts that bacteria will struggle to develop resistance to drugs that attack the public good. Imagine, for example, that researchers develop a drug that attacks siderophores. The result will be that bacteria will starve for iron.
Now imagine that a bacterium undergoes a mutation that protects its siderophores from the drug. He will have no advantage. Bacteria release all their siderophores cooperatively into the host, where the molecules mix. When a bacterium absorbs an iron-bearing siderophore, chances are it is not its own siderophore. Therefore mutants will not be able to reproduce at the expense of their friends.
Sociomicrobiologists first developed this argument abstractly using mathematical equations and computer simulations. "We develop all these theories and say, look, this should work if we just try it," Pepper says. "But all the effort will be in vain if no one tries." And such experiments are indeed being conducted now. Recently, for example, Comerly, Brown and their colleagues tested a drug that attacks siderophores. Previous studies have suggested that siderophores produced by Pseudomonas capture the metal gallium as easily as they capture iron. The researchers wondered if it was possible to use gallium as a drug that would prevent the bacteria from iron.
To test this, they conducted an experiment on larvae. They infected the insects with Pseudomonas. Some of the larvae were treated with gallium and others were left without such treatment. All larvae treated with gallium recovered, unlike the others, where the infection spread and killed them all. After showing that gallium can be used as an antibacterial drug, the scientists conducted another experiment to see if the bacteria could develop resistance to the drug. According to the theoretical considerations, they should not develop resistance. "We were concerned when we conducted the evolutionary experiment," says Comerly. He and his colleagues knew full well that other promising drugs had succumbed to the power of evolution. "We just hoped that evolution wouldn't pop up," he adds.
For their new experiment, the scientists grew Pseudomonas in a culture liquid that contained iron. But the iron was trapped in molecules that the bacteria could not absorb. To survive they needed their siderophores, which would extract the iron from the molecules. In one of the experiments, the scientists exposed the bacteria to regular antibiotics. At first, the drugs inhibited the growth of the bacteria, but after 12 days of exposure, the bacteria became completely resistant to antibiotics.
After that, they conducted the experiment again, and this time exposed the bacteria to gallium instead of regular antibiotics. The gallium slowed the growth of the bacteria considerably. After 12 days, the bacteria were just as sensitive to gallium as at the beginning. The experiment met the predictions of the sociomicrobiologists. A drug focused on the public good prevented the bacteria from developing resistance.
Pepper, who was not involved in the experiments, believes that the gallium experiment is a fundamental success for sociomicrobiology. "I think this is exactly what is needed as the next step," he says. "I hope this will be a turning point for us." Comerly hopes that other scientists will begin testing gallium in mice and perhaps, in a few years, in humans. Such experiments will be relatively easy to perform because gallium has already been thoroughly tested in humans for several medical treatments.
Potential remedies
Siderophores are just one example of a public good that may serve as a drug target. There are bacteria, for example, that make us sick by releasing toxins. But they only do this when their population is large enough to launch an effective dose of poison. So they release toxins that cause our cells to explode and feed on their contents. Medicines that can neutralize the toxins may render the bacteria helpless without even destroying them.
Other researchers are looking at the signals that bacteria send to each other. They discover molecules that can damage communication in a variety of ways, such as blocking the receptors that normally respond to the molecular signals. If bacteria can't communicate with each other, they can't cooperate.
Antisocial drugs may have another advantage over regular antibiotics: instead of killing many types of bacteria at once, they may be able to focus more on the target bacteria because a public good produced by one strain usually only benefits that strain. Therefore, antisocial drugs should not kill the good bacteria along with the bad.
However, although this approach seems promising, some scientists doubt that antisocial drugs will not induce resistance. Thomas Wood of the University of Pennsylvania and his colleagues are investigating some of the most promising compounds of this type. And their results cause disillusionment. In a trial of a drug that interferes with signaling between bacteria, for example, they discovered bacteria whose mutation allowed them to grow despite the drug. In other words, the bacteria developed a way to live without public goods. "I'm not discouraged," says Wood. "I just think that this particular class of drugs is not a panacea."
Wood's results may be due to the fact that some public goods are not truly essential. Therefore, evolution-based medicines will have to focus only on vitality. Even if antisocial drugs only slow the development of resistance, Pepper says, they will be an important advance. "We are losing the race, and lives are at stake," he warns. "Even if we only gain an advantage against the opponent, it will save many lives."
in brief
Researchers in a new field called sociomicrobiology believe they have discovered a new approach to combating the resistance of disease-causing bacteria to antibiotics. They want to interfere with the processes that allow bacteria to communicate and cooperate with each other.
The theory of evolution predicts that it will be difficult for bacteria to develop resistance against these "anti-social" drugs. But not everyone is convinced that this new approach to antibiotic development will succeed.
About the author
Carl Zimmer is a New York Times columnist and the author of 13 books, including "Evolution: Understanding Life". His latest article in Scientific American focused on the oldest rocks on Earth.
A case story
The lessons of evolutionary biology
Researchers hope to develop more effective antibiotic treatments by affecting the ways in which different bacteria communicate and cooperate with each other. Theoretically, such an approach might induce less resistance, because individual cells would not benefit from changing their way of responding. One idea is described below, which focuses on a molecule that Pseudomonas bacteria use to take up iron.
The goal: group collection of foodstuffs
Pseudomonas bacteria produce molecules called siderophores to steal iron from their host, as depicted in the blue track. Each siderophore can be recycled and used by many bacteria, and in this sense it is a public and not a private good.
The strategy: to consume the common goods
The pink route describes a treatment approach inspired by sociomicrobiology: harming the utility of the public goods. Here gallium undermines the ability of the siderophores to supply iron to the bacterial population. Even if a single cell develops a beneficial mutation in its siderophores it will die out because it will likely use the siderophores produced by other bacteria.
More on the subject
Hacking into Bacterial Biofilms: A New Therapeutic Challenge. Christophe Bordi and Sophie de Bentzmann in Annals of Intensive Care, Vol. 1, Article No. 19; June 13, 2011. www.ncbi.nlm.nih.gov/pubmed/21906350
Gallium-Mediated Siderophore Quenching as an Evolutionarily Robust Antibacterial Treatment. Adin Ross-Gillespie et al. in Evolution, Medicine, and Public Health, Vol. 2014, no. 1, pages 18–29; 2014. www.ncbi.nlm.nih.gov/pubmed/24480613
The article was published with the permission of Scientific American Israel
More of the topic in Hayadan:
- Nobel laureate Sidney Altman: "It is possible to eliminate the resistance of many bacteria to antibiotics, the pharmaceutical companies are not so cooperative"
- The US Centers for Disease Control and Prevention: We have reached the end of the antibiotic era
- Prof. Ada Yonat: "The reluctance of pharmaceutical companies to develop new antibiotics for economic reasons could set us back decades when it comes to life expectancy"
- A new type of antibiotic will eliminate bacteria that are resistant to existing drugs
7 תגובות
In simple Hebrew, they need to make their society capitalist....it will already take care of eliminating everyone 🙁
Gideon, you can remove worry from your heart and apply your method as much as you want. Evolution will simply work overtime and weed out the idiots who avoid using proper medical methods to survive and thus greatly improve the human race. All kinds of measles vaccine refusers at a young age are already on the right track. rise and succeed! (to die at a young age, meaning).
Gideon
I actually think that we should get rid of the stupid...unfortunately they have more children and thus the average intelligence decreases over the generations.
You are proof of that….
Gideon
Beyond the malice in your response and its pointlessness,
You should know that babies have a weak immune system.
If bacteria are "allowed" to kill the "weak". We are condemning the human race to extinction.
Second, compared to humans 500 years ago, we all have weak and degenerate immune systems.
The spoiled use of clean water and drugs has degenerated us all.
Until we develop nanobots that destroy bacteria this is what we have.
To Gideon, your thinking fits those who think we will be dependent on evolution forever. We are close to the point where our primary evolutionary development will depend on us and evolution itself will have a secondary role.
good
We have to let the bacteria kill weak humans, otherwise we will find ourselves in another 500 years with a degenerate immune system.
Currently, a person with a successful immune system has no evolutionary advantage over his weak friend who is treated with antibiotics.