Unprecedented abilities to produce thousands of enzymes pave the way for a green industrial revolution
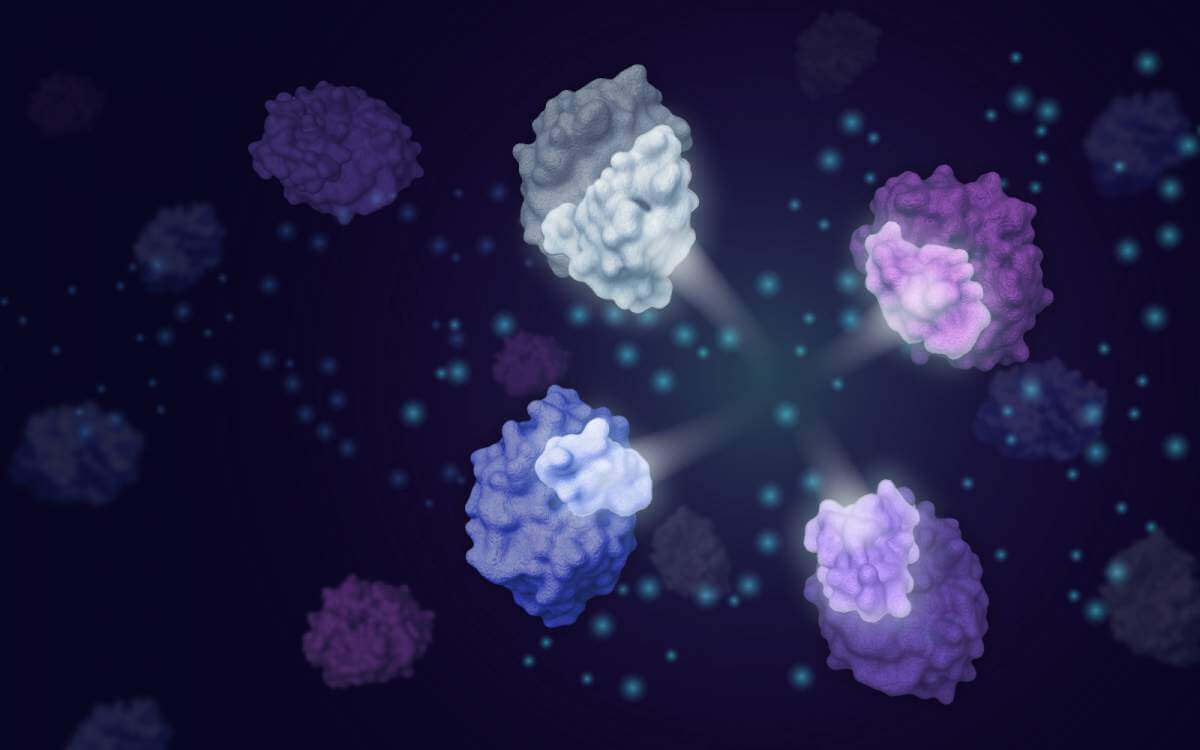
From breaking down polluting waste and purifying wastewater to creating renewable energy - custom enzymes are a promise for a greener industrial future. In a new study published today in the scientific journal Science, Weizmann Institute of Science scientists are bringing the vision closer to reality: a team of researchers led by Prof. Sheral Fleishman from the Department of Biomolecular Sciences presents a new computational method for designing enzymes by assembling them from modular building blocks. This method, which draws inspiration from our immune system, makes it possible to design thousands of active enzymes with unprecedented efficiency and is a leap forward in the field of protein engineering.
Enzymes are, without a doubt, one of the most prominent examples of the achievements of evolution. These molecular machines, without which life would not be possible, carry out all the chemical processes that take place in the living body. Millions of years of natural selection have perfected and improved their activity, so that the enzymes are able to speed up the rate of certain chemical reactions by billions and even more. In fact, the vast majority of the chemical reactions on which life is based would not occur at the rate required for life to exist without enzymes. Like other proteins, the properties of enzymes depend on the genetic sequence of the genes that encode them and the creation of a precise three-dimensional structure based on it. A mistake or a change in the sequence may result in not creating the structure required to perform the function of the enzyme, but nevertheless they may also increase its efficiency.
According to this evolutionary principle, today biochemists engineer enzymes by trial and error: first they generate random changes in the genetic code of natural enzymes, and then examine how effective the engineered products are for various purposes. Like evolution, it is a complex, slow and laborious process. In Prof. Fleishman's laboratory, they decided to do it in a different way: to break down natural enzymes into modular segments, so that, similar to Lego blocks, it would be possible to change and reassemble them in different ways and create a large number of very diverse enzymes.
This innovative approach was inspired by our immune system, which is capable of producing billions of different antibodies by randomly splicing segments based on a limited number of genes. "Antibodies are the only protein family in nature that is produced in a modular way," says Prof. Fleishman. "Their enormous diversity was created thanks to the assembly of existing genetic segments. This is similar to how a new electronic device consists of existing processors and transistors."
""We were amazed by the results. Although a success rate of 0.3% is apparently not very high, the net number of different active enzymes - 3,000 - is enormous. A typical experiment in enzyme engineering yields no more than a few dozen active enzymes"
But is it even possible to break down enzymes into modular building blocks? Rosalie Lipesh, a research student in Prof. Fleischman's lab who led the new study, set out to test the question when she was surrounded by dozens of natural enzymes that break down xylan, a common substance that, like cellulose, is found in the walls of plant cells. "The choice of enzymes that break down xylan is not accidental," she explains. "If we succeed in increasing the activity of these molecules, which are found naturally in various species of bacteria, we will be able to break down agricultural waste more efficiently and produce biofuel from it."
To test the method of modular segments, Lipash developed an algorithm capable of selecting, based on physical calculations and machine learning, the segments of the protein sequence from which the chances of assembling stable structures are the highest. Using the algorithm, the researchers broke down the natural enzyme sequences into selected segments and designed dozens of mutations in each segment designed to turn them into modular building blocks as good as possible. In the next step, the algorithm reassembled the engineered building blocks in different combinations and generated a million sequences expected to produce stable enzymes.
With a million sequences in hand, the researchers turned to practically producing a million enzymes in order to test their effectiveness in the laboratory. To their surprise, about 3,000 enzymes were found to be active. "We were amazed by the results," said Prof. Fleishman. "Although a success rate of 0.3% is apparently not very high, the net number of different active enzymes is enormous. A typical enzyme engineering experiment yields no more than a few dozen active enzymes."
When they gathered such a rich repertoire of active enzymes, the scientists raised a central question that preoccupies protein researchers: what molecular characteristics distinguish active enzymes, and distinguish them from inactive enzymes?
With the help of machine learning tools, Lipch examined about 100 properties of enzymes and selected from them ten properties that best predict whether an enzyme will be active. She integrated the "activity predictor" into her algorithm and repeated the experiment of designing the enzymes to break down xylan. The second run of the experiment already yielded about 12,000 active enzymes - 9,000 enzymes for breaking down xylan and another 3,000 for breaking down cellulose. In other words, the researchers doubled the success rate in the first experiment 10 times and led to an unprecedented achievement in the history of protein engineering: they developed more active enzymes in a single experiment than those produced over a decade.
Furthermore, the enzymes obtained were endowed with extraordinary diversity both in their sequence and in their structure - a diversity that indicates the possibility of their ability to act on other materials in addition to xylan and cellulose.
"Automatic and reliable technology for the production of enzymes with such a high level of activity is excellent news," says Lipesh. In fact, the new method, which the scientists called CADENZ - English acronym for "combinatorial assembly and design of enzymes" - is going to revolutionize the field, and it will be possible to apply it not only to enzymes but to any family of proteins. The research team in Prof. Fleishman's laboratory is already examining the application possibilities of the method to design new and improved antibodies and to create new variations for fluorescent proteins used as markers in research laboratories.
"Protein engineering is becoming a central component of the global economy and pharmaceutical industry," says Prof. Fleischman. "Our goal in the laboratory is to change the way enzymes, antibodies and other proteins are engineered - so that the process will be more efficient and reliable and pave the way for a real industrial revolution."
The research participants include Dr. Olga Khersonsky and Shlomo Yakir Hoch from the Department of Biomolecular Sciences at the Institute; Dr. Sybryn P. Schroeder and Dr. Kasper de Boer and Prof. Herman S. Overklift from the University of Leiden, Netherlands and Prof. Gideon G. Davis from the University of York, UK.