Repeated failures to develop an AIDS vaccine compound have sent researchers back to the drawing board
By David W. Watkins
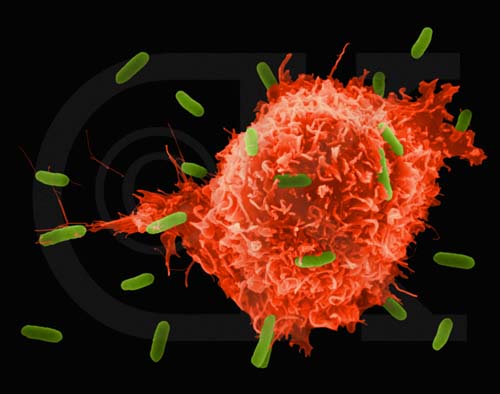
Not long after the virus that causes AIDS was identified, Margaret Heller, who was the US Secretary of Health at the time, told a group of journalists that the discovery would allow scientists to develop a vaccine component that would prevent AIDS. "We hope that such a component will be ready for testing in about two years," she announced proudly. The year was 1984.
Government officials are wrong about other matters as well, but unusually many in the scientific community were also overly optimistic. 25 years after the virus was isolated, we still do not have an effective vaccine. In 2007, large-scale clinical trials by the pharmaceutical company Merck were stopped because it became clear that the ingredient was not helpful and may not even cause harm. In the summer of 2008, another hope of finding a component was shelved and the tests were canceled before they even started because there was no reason to believe that their results would be better.
After more than twenty years of attempts to develop an anti-HIV compound, the discontinuation of the tests caused an uproar in the field. We realized that if not one of the classical methods for preparing an ingredient works against this virus, we must develop a new method. We must take an unusual and creative approach that has not been taken before or discover some new insight into the virus that will help discover its vulnerabilities. We have to go back to the starting line, but that doesn't mean we haven't learned anything in the past 25 years. In fact, each failure taught us tricks the virus uses and opened up new ways to try to attack it. These insights are already generating new ideas and encouraging scientists to work together to answer unsolved questions about this unique virus.
How do ingredients work and why are they ineffective against HIV?
In order to understand how to solve the problem of developing an anti-HIV vaccine, one must first understand how vaccine vaccines generally work. There are several methods of preparing ingredients, but the effectiveness of the ingredient always depends on the natural immune response of the human body. For example, the annual flu shot is made by neutralizing the strains of viruses that are common that year, and the dead viruses are injected into people. The cells of the immune system in the deep layers of the skin recognize the viral proteins as foreign and within a few weeks they cause the body to produce tiny molecules - antibodies - adapted to the strains of the injected viruses. If one of these viruses enters the body during the flu season, the antibodies will "neutralize" it by binding to it and thus prevent it from infecting the host's cells.
In 1962, Albert Sabin developed a successful compound against polio (polio) from live but weakened (neutralized) polio viruses. Since a live component is able to infect cells to some extent, it causes not only the creation of antibodies but also a cellular immune response by special cells called T lymphocytes. When a person vaccinated in this way is exposed to the polio virus, the T cells respond quickly and destroy the host cells that have been infected by viruses that have managed to escape the antibodies .
These two examples represent the principles underlying the main ingredients against infectious diseases in the last 50 years. Unfortunately, these standard methods of generating antibodies and T cells failed to protect against HIV. All the ingredients mimic different aspects of natural infection, and allow the immune system to produce a "memory" of the event and react more aggressively the next time. However, HIV appears to be almost completely adapted to evading the natural immune system or becoming paralyzed.
When HIV first infects a new host, it multiplies rapidly within the host's cells, and the new viruses take over additional cells [see box on opposite page]. The culture of the virus is very accelerated, and there are people who, a month after the date of infection, can find 100 million copies of the virus in a milliliter of blood fluid. Usually the first line of defense of the natural immune system is the innate, or "non-specific" immune system, which consists of cells that patrol the body and pick up invaders. Some of these cells will immediately destroy any cell infected with the virus they encounter. In most people, this system probably fails to overcome the initial attack of the proliferating HIV virus. At the same time cells of the innate immune system called "antigen presenting cells" are busy ingesting some of the viral proteins so that they can present them to more specific components of the immune system and thus trigger an immune response.
A suitable part of these are the T cells mentioned here, which are divided into two main types: "helper" T cells and "killer" T cells. The helper T cells play a vital role in sounding the alarm that activates the cellular immune system and orchestrating the attack. First, the antigen-presenting cells present to the helper and killer T cells the foreign proteins, i.e. the antigens they have sampled. The antigen-presenting cells use molecules belonging to the histocompatibility complex (MHC) to present the protein fragments. The T cells then use their receptors to recognize the MHC-protein conjugates [see figure on the next two pages]. From the moment the killer cells have a description of the invader and they receive a chemical signal from the helper cells, they multiply and go on a reconnaissance and extermination mission. This response of killer T cells occurs about three weeks after infection, it destroys most of the cells infected with the virus and lowers the level of the virus in the body to low values. But often the response is too little and too late, and in the meantime chronic infection with the virus occurs for life.
Helper T cells, often characterized by the CD4 receptor, a molecule unique to them found on the cell membrane, represent perhaps the most important system in the body for controlling responses to infectious agents, due to their central role in directing the activity of other cells of the immune system. Unfortunately, to begin with, HIV attacks the CD4 cells themselves, multiplying within them and destroying them. Within a few weeks of initial infection, the virus damages the basal pool of helper T cells so severely that the entire immune control system is damaged and never fully recovers.
At the same time, the virus perfects its ability to evade killer T cells. Its genetic material is in the form of RNA. After entering the cell, HIV copies this information into DNA. This process is carried out carelessly which causes mistakes, that is, mutations in the viral copy. These changes are passed on, and every time the offspring of the virus copy themselves, new changes are created. Moreover, if two viral copies infect the same cell, they can exchange genetic information between them in a process known as recombination, thus creating another version of the virus.
Due to this increasing diversity, the cells of the immune system, which know the original version of the virus, do not recognize the virus proteins displayed on infected cells. And because killer T cells destroy only the cells that present antigens that can be recognized, the cells infected with mutant viruses take over. Antibodies produced by the immune system three to four weeks after the initial infection cannot therefore recognize many of the viruses in the host's body in later stages.
The inability of the immune system to recognize the new and different versions of HIV is the main source of frustration for the ingredient developers, as it hinders both the function of the antibodies and the function of lethal T cells that are created following the vaccine. A strong immune response against one strain of HIV may be useless against the strain that eventually enters the body or against the mutant versions of the original virus strain.
To understand the magnitude of the challenge in dealing with the enormous diversity of HIV, it is worth remembering that the manufacturers of the ingredients change the anti-influenza ingredient every year, because the influenza viruses that are common in the world are constantly changing through slight changes in their envelope proteins. Last year's antibodies therefore fail to recognize the current flu strains and protect against them. The AIDS virus mutates so rapidly that six years after infection, the protein on the surface of the HIV particles in one person has a greater variation than the variation of all influenza strains in the world in a given year. In fact, a compound that relies on traditional methods of producing antibodies and other immune system responses against HIV would have to immunize against thousands, perhaps even hundreds of thousands, of different viruses and not just one virus.
Changing targets, chain of failures
The most effective long-term solution to the HIV epidemic would be a component that would prevent infection completely. For this purpose, at the very least, a component will be required that will stimulate the creation of broad-spectrum neutralizing antibodies that can recognize the HIV virus in all its forms and prevent it from infecting cells.
When scientists discovered that in order to penetrate helper T cells, the HIV virus must bind to the CD4 receptor and an auxiliary receptor known as CCR5, the receptors were a central target in the research of component development: the researchers were looking for a way to prevent the virus from binding to them. One of the main target molecules of the research is a glycoprotein (a protein to which sugar groups are attached), which is found on the envelope of the virus and binds to the two receptors before the virus fuses with the cell. But the variety of versions of this protein, known simply as the "envelope", is even greater than the variety of the other proteins of the virus.
One of the first anti-HIV compounds tried on humans was called AIDSVAX, and it was supposed to trigger an immune response against the envelope. After tests that began in 1998 and lasted five years, the assembly was defined as a failure. Antibodies produced by the vaccine did not prevent HIV from infecting CD4 T cells, so they did not prevent the infection of vaccinated individuals.
To date, no anti-HIV compound tested in humans has elicited the production of broad-spectrum neutralizing antibodies required to prevent HIV from entering cells. Since the problem of neutralizing antibodies was and still is the main obstacle to the development of a safe and effective ingredient, the researchers are now testing another, less desirable option of an ingredient that does not prevent infection, but reduces the risk of getting sick or passing the disease to other people.
Such a component will try to maintain low levels of the virus in the body by stimulating killer T cells to destroy infected cells, thus preventing the sharp increase in the levels of the virus in the body in the initial stages of infection. Suppressing virus culture during the initial infection phase can protect the body's T helper cell population. It can also reduce the risk of transmitting the virus to others. After the initial replication burst of the virus, HIV levels stabilize in people who are not receiving drug treatment at about 30,000 copies per milliliter of blood fluid. Observational studies have shown that the risk of people with less than 1,700 copies per milliliter in their blood passing the virus to their healthy partners is much smaller. Any anti-HIV agent that cannot prevent infection should therefore aim to limit peak levels of the virus and reduce the chronic viral load to 1,700 copies or less.
This approach is also supported by data from studies of human HIV infection and studies of monkeys infected for research purposes with the corresponding monkey virus, SIV. The data show that killer T cells are important for viral load control. Moreover, there are rare cases in which the bodies of humans and monkeys manage to control the reproduction of the AIDS virus without a vaccine or drugs. Most of these people have certain versions of genes that code for making MHC molecules that stimulate killer T cells to respond against foreign antigens.
Such evidence laid a conceptual foundation for the development of killer T-cell-stimulating compounds, and researchers had high hopes for recent clinical trials testing an anti-HIV compound designed to stimulate killer T-cells that recognize HIV. The Merck company, which developed the ingredient, invested considerable sums in research on an anti-HIV ingredient and tested many methods to stimulate killer T cells. In the end, it was decided to use adenovirus type 5 (Ad5), which causes the common cold, to insert three HIV genes into cells and make them produce the virus proteins. The immune system was supposed to think that the body was infected with HIV and develop an immune response. The selected proteins, called Gag, Pol and Nef, do not tend to vary appreciably between different HIV strains.
Unfortunately, this promising approach to stimulating the killer T-cell response has failed in human trials. Subjects vaccinated with the compound developed a relatively weak T-cell response, with a strength between 10% and 20% of the response of people infected with HIV and whose immune system manages to control the multiplication of the virus. Moreover, the cells reacted to only three regions on the viral proteins. In contrast, people infected with HIV and whose body controls the reproduction of the virus develop between three and six specific responses against the Gag protein and additional responses against other proteins.
It is possible that the failure of the Merck company was due to the use of Ad5 to insert the genes or the selection of these particular genes of the HIV virus or both. Ad5 may not be able to elicit a strong enough cellular immune response to control HIV infection. Many of us have been infected with this common cold virus, and have already developed an immune response against it. Therefore, the antibodies already present in our body will limit the number of Ad5 particles that can infect target cells and weaken the effect of the virus. It is also possible that killer T cells previously generated in the body against adenovirus took over the immune system's initial response to the compound, reducing the efficiency of the formation of HIV-specific killer T cells. It is also possible that the three HIV genes chosen to be assembled are not efficient enough to control the virus's growth rate.
Unlike the Ad5 component, which produces a limited number of HIV proteins, a live, attenuated SIV component produces all viral proteins except parts of the Nef protein. Like the polio virus, this live virus can reproduce in vaccinated monkeys, although less than the natural virus, and it protects the animals from infection with different versions of SIV, which differ considerably from the virus. Such an ability to immunize against infection, even when the immune system encounters different versions of the virus, is of course the goal of scientists trying to develop an HIV component. But the experiments also show that the weakened virus in the compound eventually manages to repair itself and cause a powerful SIV infection that kills the monkeys. Moreover, the component viruses and the viruses used to test the effectiveness of the vaccine can fuse and create a new and deadly strain. Due to these safety issues it is unlikely that live, attenuated HIV will be used as a component for humans.
the way forward
The failure of Merck's compound was a fatal blow to the field, sparking public debate over the possibility of developing an effective compound against HIV. The failure also brought about a re-examination of the ingredient candidates. Tests currently being conducted in Thailand and expected to be completed this year are the only large-scale experiments currently being conducted on humans to examine ingredients, and no new tests are expected in the near future. A large international trial of a component based on plasmid DNA developed at the US Institutes of Health (NIH) was scheduled to begin in the fall. But in July 2008, Anthony S. Posey, director of the American Institute of Allergy and Infectious Diseases, canceled the tests, arguing that the evidence did not support conducting such a large-scale trial.
At the same time, Posey announced that his office would direct the resources intended for ingredient research to a channel of basic research that would answer fundamental questions about HIV and its behavior in the body. Such research could reveal new approaches to neutralizing the virus. According to him, in order to develop the next generation of improved ingredients, scientists must deal with several important issues.
The variability of HIV is still a major barrier to the development of effective antibody or killer T-cell protection in the initial stages of infection. Due to mutations and recombination events in the body of each carrier, a vaccinated person is expected to be exposed to a virus that differs by more than 10% from the virus that was used to prepare the vaccine. For example, cumulative changes in one of the genes that undergo mutations at a rapid rate, such as the env gene that encodes the glycoprotein of the virus envelope, are important for classifying HIV into different groups (marked M, N and O) and subgroups. Analysis of the amino acid sequence of the coat protein shows that the sequence can vary by up to 35% between one subgroup and another. Even within a subgroup the envelope protein sequence variation can reach 20%.
Many of the designed components, based on killer T cells, therefore do not rely on the use of the envelope protein to stimulate an immune response. Instead the compounds target more conserved regions of the virus, such as the Pol and Gag proteins. However, it should be taken into account that a relatively small difference in these proteins may have a serious effect on the effectiveness of the ingredient. Changes of single amino acids in a viral protein can impair the ability of the antibodies or killer T-cells created due to the vaccine to recognize the virus or even eliminate it altogether. The most important goal in the field of anti-HIV compounds was and still is to produce broad-spectrum neutralizing antibodies.
Another question is related to the response of the host's killer T cells against viruses during natural infection: do we want to mimic or enhance all of them, or should we focus on certain types? Killer T cells select different parts of HIV and react to them according to the amino acid sequence of these proteins, and there are viral regions that provoke reactions more than others. It also turns out that not all the responses of killer T cells are equal in strength, and some of them are more effective than others in suppressing the virus culture. New laboratory tests recently developed are expected to help us determine for the first time which of the many responses of killer T cells can restrain the HIV virus culture in the laboratory. If it turns out that some of the rarest responses observed during natural infection are actually the most effective in suppressing the virus, then the most successful approach to developing a compound would be to strengthen these responses by tuning HIV-specific T cell responses.
Similarly, if we understand how exceptional carrier individuals known as "elite controls" are able to suppress the culture of HIV or SIV naturally, we can improve the design of compounds. Few people and monkeys manage to achieve spontaneous control of the virus culture in their bodies after infection, just as an effective ingredient that stimulates the activity of killer T cells should work. In these cases, the suppression of the virus culture occurs after the initial infection phase has passed, so the study of this transitional phase is expected to provide clues about the possible way to curb the virus. We already know that the bodies of some of these carriers have genetic changes that increase the number of immune system cells or increase their activity, or alternatively changes that reduce the ability of the virus to bind to the CCR5 receptors on the cells. These days the researchers are engaged in gathering a large group of human "elite controllers" for the purposes of extensive genetic, immunological and virological studies. It is likely that these studies will yield important clues as to the ability of these people to suppress the viral culture. These discoveries will provide new ideas for the development of ingredients, and it will be possible to test them through experiments on monkeys.
Further studies on the response of monkeys to live and attenuated SIV components would also be valuable because these powerful components allow monkeys to repel particularly virulent viruses for considerable periods of time, even those that differ significantly from the component strain. Although safety considerations will never allow the weakened virus to be used in humans, we may discover new insights if we understand why it is so effective.
Finally, a new strategy we employ in our work will help scientists discover a new approach to creating an anti-HIV compound. For the first time, groups of researchers are coming together to answer the key questions, and these collaborations are funded by the Bill and Melinda Gates Foundation, the International Initiative for the Development of HIV and the US Institutes of Health (NIH). These collaborations have a greater chance than before of finding valuable clues that will facilitate the development of the anti-HIV compound.
The developers of the anti-HIV compound are far from giving up and are preparing for a renewed fight. In Margaret Heller's day, we could not have guessed how tenaciously this virus would challenge traditional vaccination methods. But we are also stubborn, and in the course of time science will find a way to defend against HIV.
key concepts
So far, the AIDS virus, HIV, has defeated all scientists trying to develop a vaccine component because the virus evades the immune system and subverts it. If it is impossible to prevent HIV infection, the second highest goal of the ingredient developers is to reduce the spread of the virus and reduce the severity of the disease it causes. Researchers are already returning to the basic science phase to find new clues, and they are far from giving up.
The goal is an early immune response
An ideal ingredient should activate the body's immune system to prevent HIV from infecting cells. A less good solution will allow infection but will prevent the virus from multiplying at a rapid rate during the initial and critical stages of infection. In order to achieve these goals, the ingredients usually rely on stimulating some of the immune responses that arise from natural infection to create a "memory" of the virus. But the tremendous mutation rate of HIV often thwarts this approach, because the range of immune memory is not broad enough. To develop an effective compound, it is necessary to produce antibodies and lethal T cells capable of recognizing the different HIV particles to 20% of the version that was used to prepare the compound.
A few hours after entering the body, the HIV virus infects T cells that carry the CD4 receptor, and begins to replicate rapidly. Antigen-presenting cells (APCs) pick up foreign invaders and engulf infected cells when they encounter them.
After a few days, APCs present small fragments of the virus on molecules that belong to the tissue compatibility system (MHC). The fragments are presented to uninfected CD4 helper T cells and killer T cells. In response, helper T cells release chemical signals that activate B cells and killer T cells, and these begin Some of these B and T "memory" cells remain in the immune system to respond to future infections.
Within a few weeks, killer T cells will hunt down infected cells and display viral antigens on the cell membrane to destroy them, while the antibodies prevent the virus from infecting new cells.
The goals of the composition
To induce the production of B cells and T cells capable of responding to HIV, compounds attempt to simulate infection of the host cells by HIV. Most of the currently developed components package HIV genes inside a live and harmless virus or bacterium or inside a DNA ring known as a plasmid, which can enter the host's cells. When the cells produce the viral proteins encoded by the genes and display them, the immune system recognizes them as infected cells.
An alternative plan to assemble
Changing the course of infection
Usually in the first 21 days after infection, HIV destroys many helper T cells, which are supposed to coordinate the immune response. This cell population never fully recovers from the attack. Any component that is unable to prevent infection should focus on maintaining low levels of the virus in the body from the initial stages. Saving helper T cells may prevent the sharp decline in the overall efficiency of the immune system, which ultimately leads to AIDS, that final, multi-symptom stage of HIV infection.
Essential hints
Rhesus macaques are a valuable model for AIDS researchers because they are susceptible to the SIV virus, which is very similar to HIV. Compounds made with attenuated versions of SIV completely protected the monkeys for years against SIV infection. Unfortunately, the weakened strain eventually repairs itself and the monkeys contract AIDS caused by the compound. If we understand why the compound protects the monkeys for such a long time, we can find out what immune responses must be induced to develop an effective compound.
Timeline of the ingredients
In the last decade, only a handful of ingredients managed to reach the stage of large-scale clinical trials in humans. Until now, traditional methods for developing compounds, which try to stimulate the creation of antibodies or the mobility of T cells, have failed to yield the protective compound against AIDS. Due to these disappointments, the American Institute of Allergy and Infectious Diseases (NIAID) announced in the summer of 2008 that it would direct its resources to basic research on HIV.
1984
On April 23, Margaret Heller, the US Secretary of Health, and Robert Gallo, head of the Department of Cell Biology of Tumors at the American Cancer Institute, announced the discovery of the virus that causes AIDS at a press conference. Following the discovery of the infectious agent, Keller said that the anti-HIV compound will be ready for clinical trials in about two years. New HIV particles sprout from the surface of an infected helper T cell
1999
VaxGen's AIDSVAX component was the first to reach Phase III clinical trials. In 2003, after trials in the United States, Canada, the Netherlands, Puerto Rico and Thailand, AIDSVAX, designed to stimulate the production of antibodies to the outer envelope of HIV, was declared a failure. The compound did not provide better protection against infection compared to a dummy vaccine (placebo).
2003
The United States and Thailand have begun a large-scale trial of a compound designed to stimulate a T-cell response to the envelope protein by priming the immune system with the canarypox virus. Many scientists raised their voices against the experiment at the very beginning because weak reactions to the compound were seen in small-scale studies. Final results are expected in 2009.
2004
Greater hopes accompanied Merck's STEP tests, which tested a component in which three HIV genes were integrated into the Ad5 virus that causes the common cold. This component was also designed to stimulate T-cell protection, and it caused a strong immune response in the recipients of the component. However, the trials were stopped in 2007, when follow-up of the volunteers showed that vaccine recipients contracted HIV more often than placebo recipients. The reasons for the component failure are still being investigated.
2008
International trials of a compound that inserted HIV genes packaged into exposed DNA and then into Ad5, which had been scheduled to begin in September, were canceled in July by NIAID Director Anthony S. Posey. In these tests, called PAVE 100, 2,400 men were supposed to be tested. In more limited tests, it was found that the immune responses after the administration of the component were not significantly different from the immune responses observed after the administration of the soup component, and Fossey declared that the scope of the planned tests was not justified.
The author
David W. Watkins (Watkins) researches the biology of the immune system at the University of Wisconsin-Madison, where he also directs a molecular diagnostics laboratory for the university hospital and its clinics. He specialized in HIV and AIDS research, won an Elizabeth Glaser grant, and served on a subcommittee of the American Institutes of Health (NIH) for the study of vaccine components against AIDS. In his laboratory in Wisconsin, he developed a program to study SIV, the AIDS virus of monkeys, in the hope of better understanding the immune response of humans to HIV and how to develop an effective compound.
And more on the subject
HIV Pathogenesis: The First Cut Is the Deepest. Louis J. Picker and David I. Watkins in Nature Immunology, Vol. 6, no. 5, pages 430-432; May 2005.
Basic HIV Vaccine Development. David I. Watkins in Topics in HIV Medicine, Vol. 16, no. 1, pages 7-8; March/April 2008.
HIV Vaccine Research: The Way Forward. Anthony S. Fauci et al. in Science, Vol. 321, pages 530-532; July 25, 2008.
Reflecting on a Quarter Century of HIV Research. Andrew E. Armitage, Andrew J. McMichael and Hal Drakesmith in Nature Immunology, Vol. 9, no. 8, pages 823-826; August 2008.
10 תגובות
Isaiah:
1. No one claimed you were just talking like that.
2. What you presented in your last response is different from what you suggested in your previous responses (a harmless variant of the virus)
3. This is about a vaccine and not a competition with the virus - the whole idea is fundamentally different!
AIDS vaccine for monkeys raises hopes for humans - May 18, 2009
Eli Leon and the news agencies
American researchers believe that they have succeeded in developing a new method that may lead to the creation of a vaccine for the AIDS virus. The researchers inserted an artificial segment of DNA into a virus that is harmless to animals and humans that causes the production of artificial antibodies. The engineered virus was injected into nine laboratory monkeys that were infected with monkey AIDS. It turned out that six monkeys were not infected with the virus at all and the remaining three did not develop AIDS. Despite the success in laboratory animals, the evaluation It is because in just a few more years they will be able to engineer an experimental version for humans.
________________________________________________
(Isaiah never just speaks like that)
The virus is obliged to fit spatially in order to bind to the CD4 and CCR5 sites
Therefore, these areas must be reserved otherwise he will not be able to create an attachment for the purpose of entering the cell
Therefore it is possible to take advantage of the regularity of these sites for the purpose of vaccination
Obviously, if that were the case, there would already be a vaccine, but I still haven't been able to understand how the virus can change its attachment sites and adapt to them
Michael R. - I realised. Thanks ):
Isaiah:
It's something else.
Here they created a version very similar to the original virus to infect animals.
This version - as expected - also causes high morbidity - something that is useful for the experiments that are intended to be conducted on those animals.
Your proposal in response 2 is problematic for several reasons.
You are proposing, in effect, to infect the people with a harmless virus that will successfully compete with the original virus.
This means that you are introducing another type of virus that will not be spoken by our immune system.
This is a very dangerous thing in advance - the very fact that our bodies are busy producing the replacement virus in the necessary quantities instead of their normal work is certainly not beneficial to the body.
It is even more frightening when it comes to a variant of HIV that will undergo many mutations (to continue to compete with normal HIV) and will easily become lethal even if its very ability to compete with HIV and create large amounts of copies of itself is not fatal in the first place.
Further to my 2nd comment, take a look at this (in my humble opinion this is in the general direction) -
http://www.pnas.org/content/early/2009/02/27/0812587106.abstract
how?
Why do you think they would be better than genetically engineered killer T cells?
Using smart nanotechnological robots could be the solution to AIDS.
What about Hindus the genetic material of the virus itself? Is there a practical possibility of researching the creation of a harmless variant of the virus, which will "compete" against its deadly brother, and simply overtake it and plug "holes" in the body's proteins before the harmful virus does?
Isn't this the copy of the first article?
By the way, there are once again problems if "for more articles"