On the history of crystal science from the beginning of mankind to the discovery of Prof. Dan Shechtman
Dana Ashkenazi and Noam Eliaz Galileo
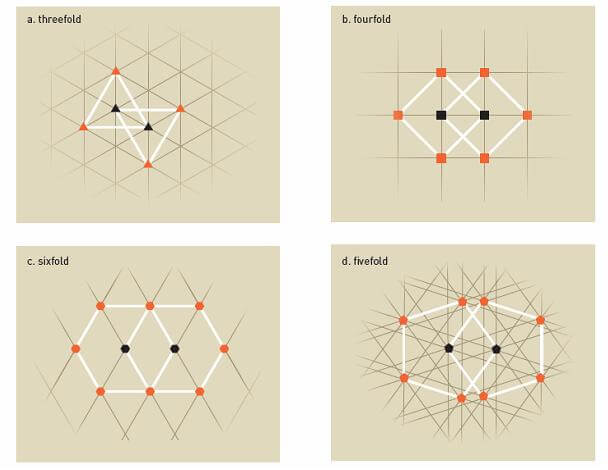
Since the dawn of mankind, man has been interested in crystals, due to their magical beauty, their impressive shapes and their multitude of colors and shades. Precious gems such as diamond, sapphire, ruby and emerald (emerald) are crystals, in which there is a long-term cyclical arrangement of the atoms of the material, which gives them characteristic properties. In ancient times, people believed that the various gemstones had magical powers that could endow their owners with virtues such as wealth, health and wisdom, and some believe this even today. In Pharaonic Egypt, the blue stone (lapis lazuli), whose color is blue stained with gold, was one of the most expensive gemstones.
This stone, which was considered the stone of the gods, was set in precious jewels. It should be noted that blue is a rock composed of several types of minerals. The ancient Egyptians also used to grind the blue stone into powder, mix the powder with water and use it for makeup. The high priest (Exodus, chapter XNUMX) used to wear a vest, in which a dozen gems were set, as many as the tribes of Israel.
This ritual accessory was placed on the priest's chest while he was engaged in the holy work in the temple. For thousands of years man has flaunted jewelry set with crystalline and sparkling gems. Men and women gave their spouses crystals as a symbol of their eternal love, and quite a few struggles and wars took place around those crystals with fascinating shapes.
From ancient Greece to the science of crystallography, the mystery of the crystalline structure has occupied the human race since ancient times. The ancient Greeks believed that all substances in the world are made of four elements: earth, water, air and fire. The Greeks believed that the crystals were made from the element earth. John Duns Scotus, a theologian of the 13th century, believed that crystals live and grow like plants, and that their structure stems from the desire of living beings to arrange themselves around an "ideal" form. The German astronomer and mathematician Johannes Kepler, known for formulating the laws of "celestial motion", was interested not only in the stars, but also in the structure of crystals. In his treatise "The Hexagonal Snowflake", Kepler claimed that snowflakes are made up of tiny spherical particles.
The Danish geologist Nicholas Steno claimed that the growth of a body - vegetative or inanimate - results from the accumulation of particles secreted from the liquid. Steno believed that an internal force is responsible for the growth of plants, while an external force is responsible for the growth of crystals. Steno did not know how to explain what that force is, but he believed that it is a small "seed" in the liquid that starts the crystal growth process by attracting particles from the liquid.
Robert Hooke, one of the greatest scientists in the 17th century (Hooke's law, named after him, describes the elongation of an elastic body in direct proportion to the force acting on it; he also coined the first biological term "cell"), followed in Kepler's footsteps, and showed that by densely compressing spheres many crystalline forms can be obtained. Since the pioneers of crystallography dealt with impure materials, which contained many defects, sorting crystals was an extremely complicated task. Therefore, for many years the field of crystallography was shrouded in darkness, until Avraham Werner (Werner), a German researcher in the field of mineralogy (the science of minerals), proposed a way to sort minerals.
Minerals are natural inorganic solid substances, usually crystalline, with defined physical and chemical properties. Werner, who was a practical man, proposed to divide the crystals into groups according to their visual characteristics. With this simple and practical approach, Werner sorted a wide variety of crystalline minerals according to their color, their degree of roughness, their specific gravity, their smell, their transparency, the way they break into pieces, their hardness, etc. Werner cataloged according to these properties every mineral that fell into his hands, thus creating a "field guide" for identifying minerals.
One of the most important breakthroughs in the study of crystal structures took place in 1845, when the French physicist Auguste Bravais was able to predict 14 possible basic combinations of geometric structures and the atoms embedded within them, from which the various crystals are built. These structures are known today as "Bravais Lattices".
Barva knitting - the three three-dimensional patterns in the illustration, based on two different geometric structures (cubic and hexagonal), represent the building blocks of some of the crystals. The red balls in the figure indicate atoms. Filling the space using any of these patterns will allow the creation of a crystalline structure. The cubic structure is a relatively simple example, since all the axes are equal and all the angles are right. Most of the pure elements in the state of a crystalline solid have a face-centered cubic, body-centered cubic, or hexagonal structure
X-rays
Crystallography (the theory of crystals) is a science that deals with the study and interpretation of the crystalline structures and experimental methods to determine them, including the way crystals are formed and their properties. The big breakthrough in this field took place at the beginning of the 20th century, after the discovery that it was possible to decipher the structure of crystals using X-rays.
Much of what we know about the arrangement of crystals is the result of studies conducted in the field of X-ray diffraction. X-rays are electromagnetic waves with a wavelength of 0.02-100 Å (according to the formula: Å = 10-10 m). Diffraction occurs when a wave encounters a series of obstacles with a fixed spacing between them that: (1) are capable of scattering the wave, and (2) that the spacing between them is of the same order of magnitude as the incident wavelength.
Visible light has a wavelength of 3,800-7,800 Å, that is to say, much higher than what is needed to characterize crystalline structures. In contrast, X-ray diffraction uses a beam with a characteristic energy of 1-120 keV, which yields a wavelength of 0.1 Å to a few angstroms (for the upper or lower energy limit, respectively). These wavelengths are of the order of magnitude of the lattice parameters, therefore using X-rays allows the structure of crystals to be deciphered.
In 1912, the German physicist Max von Laue irradiated a copper sulfate crystal with X-rays. In a series of experiments, Laue found that the X-rays are refracted as they pass through the crystal, and that the X-rays are electromagnetic radiation with short wavelengths, on the order of magnitude of the spacing between the crystal atoms. As a result of the irradiation of the crystal, Lava received a pattern of dots (spots), which resulted from the way the X-rays were refracted and returned from the atoms of the crystal arranged in a periodic manner.
According to the intensity of the radiation reflected from the crystal, Lava deduced the directionality of the crystal's atoms. Lava used the X-rays to obtain a "picture" of crystalline materials according to the way the X-rays refracted through these materials and proved that the atoms of the crystal are arranged in a cyclic manner. For this discovery in the field of X-ray diffraction in crystals, Eva was awarded the Nobel Prize in Physics in 1914.
The interference phenomenon
Interference is a phenomenon that characterizes wave behavior: when several waves pass through one point in space, the amplitude of the wave at that point will be an algebraic sum, taking into account the direction, of the amplitudes of all the waves at that point. This scheme of the waves is called superposition. Constructive interference occurs between waves when their maximum (and minimum) points are obtained together, i.e. when the frequency and incidence of the waves are equal. On the other hand, destructive interference occurs when two waves have the same frequency, but have an amplitude difference of half a cycle.
Lava encountered quite a few difficulties in deciphering the images he received. These difficulties came to an end in 1914, when two English scientists, William Henry Bragg and William Lawrence Bragg, found that the arrangement of the crystal atoms creates parallel planes with the same distance between them. The Bragg father and son came to the conclusion that for a given distance between the atoms and a known wavelength, there is an angle that will give maximum radiation reflection (constructive interference). Therefore, by irradiating the crystal with X-rays and measuring the angle at which the maximum reflection is obtained, it is possible to calculate the distances between the atoms of the crystal. For this discovery, the two researchers were awarded the Nobel Prize in Physics in 1915.
The founders and pioneers of the science of crystallography
The crystal puzzle
When we look around us, we see everywhere a huge variety of materials. What all these materials have in common is that they are made up of atoms (or ions) of elements. A block of iron is made up of atoms of the element iron, a block of titanium is made up of atoms of the element titanium, while the substance called water is made up of atoms of the elements hydrogen and oxygen. The elements of which the material is composed, the nature of the bonds between their atoms, the arrangement of the atoms in space and the type of defects present in the material are responsible for the properties of the material.
There are several types of bonds between atoms, including ionic bonds, covalent bonds (sharing electron pairs between atoms), and metallic bonds. In the metallic bond, the atom "gives up" electrons in its outer shell, which it "contributes" to a cloud of free electrons inside the material. This structure gives metals properties such as luster and conduction of heat and electricity.
In addition to the ionic, covalent and metallic bonds, there are also secondary, weaker bonds, called van der Waals bonds and hydrogen bonds. Van der Waals bonds are bonds formed as a result of asymmetry in the electron orbits in the outer shell. Because of this asymmetry, a certain region of the molecule temporarily becomes slightly negative, and another region slightly positive. Between such temporarily charged regions, a weak electrical attraction is created, called a van der Waals bond. For example, between the layers of atoms in graphite there are weak van der Waals bonds, which can be easily broken. Indeed, this is what we do when we write with a pencil.
Hydrogen bonds result from the fact that in certain molecules, for example water molecules, there are hydrogen atoms with a partial positive charge and other atoms with a partial negative charge. In adjacent molecules of this type, the negatively charged region of one molecule will be attracted to the positively charged region of another molecule. A substance in a solid state can have a long-term periodic arrangement of the atoms, i.e. have a crystalline structure, or have only a short-term atomic arrangement, similar to the liquid structure. In the case of short-range order, we say that the structure is amorphous, or glassy. It is possible to refer to the glass structure as a structure of a liquid that has frozen without crystallizing. In the figure you can see the difference between an amorphous structure and a crystalline structure.
As in Asher's paintings
The crystalline arrangement is reminiscent of the way bees build honeycombs, which contain a huge number of hexagons, or the way the famous Dutch artist Escher drew various motifs (such as fish, butterflies and lizards) that repeat themselves in an orderly and cyclical structure. A crystalline structure is characterized by the fact that one or more atoms (motif) can be associated with each lattice point, and there is a periodicity of arrangement in the volume of the material.
When describing the crystal structure, it is customary to refer to atoms or ions as hard spheres. As mentioned, the basic cell of the crystal is called a unit cell. The lattice is built from a collection of cyclically repeating unit cells, with some motif, one or more, located at fixed points in the lattice. The basic geometry of the unit cell can be, for example, a cube.
If the particles that make up the crystal are metal atoms, with a metallic bond between the atoms, then the crystal is a metallic crystal. If the particles that make up the crystal are ions and there are ionic bonds between them, the crystal is an ionic crystal. Many ceramic materials have such a structure. Table salt is also an example of an ionic substance with a crystalline structure, as you can see in the figure in front of you.
Cyclic arrangement of the sodium and chlorine ions in the crystal structure of table salt (NaCl). The blue balls indicate negative chlorine ions, while the purple balls indicate positive sodium ions. The crystal structure in this case should ensure both electrical neutrality and efficient packing of two types of ions of different sizes. In addition to metallic and ionic crystals, there are also molecular crystals, in which the motifs located at the lattice points are molecules, and not individual atoms. The bonds within the molecules are strong covalent bonds, while between the molecules there are weak bonds (van der Waals bonds and hydrogen bonds).
איך זה עובד?
A material made of only a single crystal is called a single crystal. In the microelectronics industry, a huge single crystal of silicon (Si) is used, which is cut into thin slices (wafers). At the end of the production process, each such slice contains thousands of components, which are used to build printed circuits. Most crystalline materials are not single crystals, but consist of a large collection of crystals growing from different solidification centers and organized in different crystal orientations. A substance containing a large number of crystals (nuclei) arranged in different directions is called polycrystalline.
The process of creating crystals is called crystallization. During the solidification of a polycrystalline material, small crystals, called embryos, are formed in different places in the liquid. These crystals grow by picking up atoms from the liquid environment. The meeting surface between two different grains is called a grain boundary. There is no perfect crystalline order at the boundaries of a nucleus, and they contain a high concentration of defects (that is, areas of disorder in the atomic packing), such as foreign atoms (impurities), which are concentrated at the boundaries and move through them quickly, relative to the speed of their movement through the volume of the nuclei.
The properties of materials are affected by the way the atoms are arranged within the nuclei and at the boundaries between them. For example, the smaller the grains of a polycrystalline material, the more grain boundaries there are, and as a result the material's strength and hardness increase (as long as the temperature is not very high). Also, there is a difference in properties between monocrystalline, polycrystalline or amorphous material.
Ceramic materials and polymers in an amorphous or monocrystalline state tend to be transparent to light, and in contrast, the same materials in a polycrystalline state tend to be opaque to light, and sometimes have a shine and reflect light similar to a mirror. There are also ceramic materials and polymers with only short-term sealed order; These materials are called glassy materials, or amorphous materials, and their properties are largely different from those of crystalline solids.
The solidification of a substance from a liquid state to a polycrystalline solid. Usually, the crystalline order is preserved in a certain volume in the material, and next to it is another volume with a crystalline structure arranged in a different direction (yellow areas in the figure). Between the ordered clusters, called nuclei, there is a disordered buffer zone, called the nucleus boundary.
polymorphisms
Polymorphism (Greek - "multiformity") means that a substance appears in different crystalline structures under different conditions of pressure and/or temperature, without any change in its chemical composition. In pure elements, this phenomenon is also called allotropy. Many ceramic materials, such as silica (SiO2), are polymorphic. The phenomenon of allotropy in iron, which is the main component of steels (iron-carbon based alloys), makes it possible to create steels with different crystal structures and different properties through different thermal treatments. This is one of the reasons why, over the years, steels have become the most common structural materials.
When pure iron is heated from room temperature, it undergoes two allotropic transitions before it melts and becomes a liquid at a temperature of 1,538 Celsius. At room temperature, iron has a body-centered cubic structure (known as ferrite), is magnetic, and can dissolve a small amount of carbon atoms in it. at a temperature of 912 Celsius. The iron undergoes a structural change (alotropic transition), and forms a cubic structure centered on a phase (known as austenite), which is not magnetic and can melt a much larger amount of carbon atoms inside it. This structure is stable up to a temperature of 1,394 Celsius, where a second allotropic transition occurs, ending in the formation of a magnetic, body-centered cubic structure, known as delta-iron. Ferrite and delta-iron have a similar crystal structure, but ferrite is softer and can dissolve a lower concentration of carbon in it.
Another important example is the element carbon, which can, in the solid state, exist as graphite (hexagonal structure), as diamond (cubic structure), as a C60 ball containing 60 carbon atoms (fullerenes, or buckyball), as well as in the form of nanotubes. In each of these materials, which are all made of pure carbon, there are different bonds between the atoms, and as a result they are characterized by different appearances and different properties.
Everything you wanted to know about diamonds
The white diamond is a precious crystalline mineral, composed only of carbon. It is a natural treasure, first among gems. The diamond is formed in nature in the depths of the earth (150-450 kilometers, or even more), where conditions of high pressure (about 40,000 atmospheres) and high temperatures (1,000-1,200 degrees Celsius, approximately) prevail. Apparently, the diamonds are formed from magmas rich in carbon (carbonate). Once formed, the diamonds may remain in the Earth's mantle for a long time, until they are brought to the surface in eruptions of magma, called kimberlite. As the kimberlite weathers, the diamonds are washed away in streams, sometimes forming secondary deposits within the drift.
Although diamonds are not energetically stable under standard environmental conditions, luckily their disintegration process is very slow, so we get to enjoy them. Artificial diamonds can be produced in the laboratory using plasma waves at high pressures and high temperatures. In this process, small pieces of natural diamonds are used, which are growth centers. Despite this, as of today they have not been able to produce artificial diamonds that compare in their cleanliness, transparency and size to natural diamonds.
The diamond is, as mentioned, one of the polymorphs of carbon. The diamond crystal consists of a cubic unit cell, in which each carbon atom is covalently bonded to four other carbon atoms. In this way tetrahedra of carbon atoms are formed. This structure, whose unit cell is shown in the figure, is called a diamond structure, and is also typical of other elements, such as silicon and germanium (Ge).
It is a very stable structure (that is, with a low energy level), because the pairs of electrons involved in the covalent bonds are as far apart as possible. It is the stability of the structure that gives the diamond its high strength and hardness. On a scale of 1-10 (1 being the softest, 10 being the hardest), proposed by the mineralogist Friedrich Mohs (Mohs) to describe the scratch resistance of various minerals, diamond has a hardness of 10, the highest of all substances in nature. Therefore, it can be used to process other materials, such as cutting, grinding and polishing.
Did you know that graphite and diamond are made of the same material?
Graphite is another polymorph of the element carbon. But unlike diamond, graphite is made up of layers containing hexagons of carbon atoms, as shown in the figure. Within each such layer, the carbon atoms are bound together by strong covalent bonds, where each carbon atom in the plane is bound to three other carbon atoms located near it. The layers are connected to each other by weak van der Waals bonds.
These weak connections between the layers make graphite soft (unlike diamond, the hardness of graphite is about 1-2 on the Moss scale), and allow it to be used (mixed with other materials, such as clay) for the production of pencils. Additional uses of graphite: making steels, rods in nuclear reactors, and reinforcing components in composite materials.
Graphite is made up of hexagons of carbon atoms, linked together within the plane by strong covalent bonds. Each carbon atom, represented in the figure as a blue sphere, is bonded to three other carbon atoms within the plane. The layers are connected to each other by weak van der Waals bonds, which make the graphite soft, and allow it to be used to make pencils.
The C60 molecule (Buckminsterfullerene), made up of sixty carbon atoms, forming a closed structure. The blue balls in the picture represent the carbon atoms. The molecule consists of 20 hexagons and 12 pentagons. The structure is identical in shape to football
Another form of carbon is discovered
In 1985, another form of carbon was discovered, called fullerene. It is a carbon molecule (covalent bonds) with a hollow spherical shape (a closed hollow structure called a geodesic structure). The discovery of this form, which exists in soot (even in the soot of a wax candle, tiny amounts of fullerene can be found) and in interstellar space, earned its discoverers, Robert Curl, Harold Kroto and Richard Smalley, the Nobel Prize in Chemistry for the year 1996.
Buckminsterfullerene, or carbon 60 (there are 60 carbon atoms that make up this molecule), is the first fullerene discovered in the fullerene family, which are substances with a molecular crystalline structure. With the passage of time, additional carbon molecules were discovered that also belong to the family of polar substances. All fullerenes consist of a combination of hexagonal rings (hexagons) and pentagonal rings (pentagons).
The Buckminsterfullerene molecule is named after the well-known architect and mathematician Richard Buckminster Fuller (Buckminster "Bucky" Fuller), who presented the American Geodesy pavilion structure he designed at an international exhibition in Montreal as early as 1967. Because the C60 molecule has a shape like that of an English football, the nickname stuck to this molecule "Bucky Ball".
There is a molecular crystal in which the motif located at the lattice points of a face-centered cubic cell is fullerene molecules, not individual atoms. Fullerene crystals can be produced in the laboratory, for example by passing a strong electric current between two graphite electrodes that are in an atmosphere of noble gases. In recent years, research and development in the field of fullerenes has expanded and progressed to a great extent, and it is likely that this field will continue to develop in the future as well.
Carbon nanotubes
Carbon nanotubes are a kind of elongated fullerene. They are obtained by folding a single plane of graphite and adding a polar hemisphere on each side of the cylindrical structure, so that a closed hollow structure is created. These nanotubes consist of a hexagonal carbon surface, similar to graphite. However, unlike the structure of graphite, in nanotubes there are also pentagons, and sometimes even saturated ones consisting of carbon atoms, which prevent the surface from being planar.
This additional structure of carbon, first discovered in 1991, does not exist in nature, but is artificially produced. Carbon nanotubes have unique properties, resulting from their unique structure. They have good electrical conductivity, and there is a rare combination of high strength on the one hand, and excellent flexibility on the other hand.
Possible future uses of nanotubes include precision electronic circuits, high-resolution television screens, medical uses (for example, to destroy cancer cells in a targeted manner), bridges, extremely light and strong car chassis, and aircraft parts.
Biomineralization
Crystals and crystallization processes also exist in the living body. The main inorganic component of bone is a mineral called apatite. This mineral belongs to the Calcium Phosphates family. In their synthetic form, ceramic apatite minerals encourage the construction and adhesion of hard tissues, such as bone. Therefore, for about 30 years some of the manufacturers of orthopedic and dental implants have been coating their products, which are basically made of metals such as titanium and its alloys, with synthetic apatite coatings.
These coatings improve the fixation of the implant by creating bony tissue around it, while preventing the formation of weak fibrous tissue in the implant/bone interface area. In the Biomaterials and Corrosion Laboratory at Tel Aviv University, to which the authors of this article belong, various processes that affect the interaction of bone-building cells with the surface have been studied in recent years. Microscopic images are presented here, illustrating the surface morphology of synthetic hydroxyapatite coatings, produced by an electrochemical process.
Israel on the map
In 1984, there was a big uproar in the world crystallography community, when an Israeli researcher from the Technion's Faculty of Materials Engineering, Prof. Danny Shechtman, together with his colleagues, published an article discussing crystals with "forbidden symmetry". Shechtman and his colleagues discovered the phenomenon as early as 1982, but due to the doubts of various researchers in the scientific community, it took about two years for the article to be published in a journal.
The crystals discovered by Schechtman and his partners, known as quasi-crystals, do not obey the laws of "classical crystal symmetry", which dominated the field of crystallography for many years. According to classical crystallography, a "crystal" is defined as a three-dimensional arrangement of atoms with translational periodicity along its three main axes. The two-dimensional space can be fully tiled with square, rectangular, triangular or hexagonal tiles.
This follows from the fact that these polygons have vertex angles equal to whole quotients of 2p. A combination of 2nd, 3rd, 4th, and 6th order rotation operations (i.e., rotation at a rate of 2p/2, 2p/3, 2p/4, and 2p/6, respectively) with 14 barva lattices yields 230 space groups .
In contrast, quasi-crystals embody a new kind of order, between crystalline and amorphous. Quasi-crystals are defined as non-crystalline materials with perfect long-range order, which lack copy periodicity in three dimensions. The first part of this definition is expressed in the appearance of sharp diffraction points, and the second part in the existence of rotational symmetry that is not according to classical crystallography (ie, rotational operations of order 5 or order higher than 6).
Rotation operations of order 5 or order higher than 6 do not allow cyclic tiling of the space. In pentagonal tiles, for example, the vertex angles are equal to 108°, a value contained 3.333 times in 2p. Therefore, around a given 'lattice point' only three pentagons can be placed, creating an angular spacing of 36°.
The discovery of Shechtman and his colleagues broke the conventions in the field of crystallography. In its wake, hundreds of new crystals were found, which until then were considered impossible. It was also found that the quasi-crystalline structure affects the properties of the material. For example, quasi-crystals are poor conductors of electricity and heat, and have a low coefficient of friction, high hardness and high resistance to welding (corrosion).
Sipa
Since the dawn of mankind, man has shown an interest in crystals and their structure. However, only in modern times did researchers such as Barva, von Lava and Bragg make scientific breakthroughs in the field of crystallography. The crystals are characterized by a three-dimensional long-range periodic arrangement of atoms, ions or molecules. This arrangement affects the properties of the material, whether it is synthetic (for example, steel) or whether it is natural (for example, bio-minerals or diamond). Sometimes, the crystalline structure of a given material changes as a function of temperature and pressure, a phenomenon known as polymorphism, and therefore the properties of the material also change.
Israel has its own contribution to the theory of crystals - the discovery of quasi-crystals with "forbidden symmetry" by Prof. Danny Shechtman and his colleagues. It can be expected that the breakthroughs in the field of crystal theory will continue and lead to the mapping of new materials and important technological developments.
6 תגובות
Does it depend on the size of the gems to have an effect?
Magnets will also be "dealt with" at some point. I made a note to myself 🙂
monument
And what about magnets, the "sucking stone"? Nothing, don't they have a right to exist among the crystals that have an effect on the body and mind?
Camila, I completely agree with you.
I don't think there are any other ways to "know" than using scientific tools. It can be said that intuition, inspiration and creativity are also relevant, but in my view these are only generators of ideas that need to be tested. Of these, probably 99% will prove to be wrong and only 1% will survive the test of reality.
I call it "sharp thinking". A combination of:
1) Awareness of the many traps our brains fall into
2) A balanced combination of openness and skepticism
3) Testing against reality - as a top value
Regarding "it will not help, it will not harm" - I think that the blurring between knowledge and belief, between illusions and reality is an unhealthy blurring. It is easy to cross borders without noticing. This begins to show its signs when decisions are made based on illusions and not based on evidence, and worse - taking responsibility for others (for example children) in decisions based on illusions. But this is not the place to elaborate on that.
You are welcome to follow my blog. I will write about it in the future (best to sign up for email updates).
monument,
I really liked the sentence you wrote in the blog you linked to:
"I'm not looking for scientific answers, I'm looking for correct answers. Science is just a tool to achieve them."
And I would like to add: science (through the scientific method) is the only tool that enables an orderly and reliable arrival at the correct answers, and it is also the only one that has proven and continues to prove its level of accuracy and capabilities through the predictions it enables (for example, through technology). Your words imply that there are also other such tools, but I am not aware of anything like that, I do know claims that other tools exist, but these tools have never passed simple tests to check their reliability (even regardless of the mechanism that underlies them - a problematic issue in itself) between Either because the people dealing with them were not interested in testing their readiness or because they took the test and failed miserably. And back to the crystals, I remember an information sheet for the mother in which it was written in the recommendations for the equipment for the delivery room bag to bring such and such crystals, and in parentheses it was written: "If it will not be useful, it will not harm", but unfortunately I cannot agree with this statement, because the lack of use in such cases does not mean the lack of harm , precisely because things do come at the expense of one another, precisely because clinging to useless tools leads to neglecting the truly relevant tools.
So much for a scientific review of crystals.
As it was said at the beginning of the article: "In ancient times, people believed that the various gemstones had magical powers that could endow their owners with virtues such as wealth, health and wisdom, and some believe this even today."
So that's exactly what I tried to check not long ago. Are there studies that prove the effects of crystals on people?
You are invited to read the results of the (somewhat delusional) journey here:
http://wp.me/p1K6uX-9E